Serviços Personalizados
Journal
Artigo
Indicadores
-
Citado por SciELO
-
Acessos
Links relacionados
-
Similares em SciELO
Compartilhar
Portugaliae Electrochimica Acta
versão impressa ISSN 0872-1904
Port. Electrochim. Acta vol.33 no.2 Coimbra mar. 2015
https://doi.org/10.4152/pea.201502125
Insights into the Electrochemistry of Deposition of Boron from KCl-KF-NaBF4 Melt
Rahul Pala,* and S. Anthonysamyb
a Department of Chemistry, School of Chemical Sciences & Pharmacy, Central University of Rajasthan, Bandarsindri, Kishangarh 305 817, India
b Materials Chemistry Division, Indira Gandhi Centre for Atomic Research, Kalpakkam 603 102, India
Abstract
Electrochemical reduction of boron from boron containing fluoroborate species present in KCl (81.54 mol%)-KF (18.45 mol%)-NaBF4 (1.67 × 10-4 mol cm-3) melt on a platinum electrode was studied by cyclic voltammetry and chronoamperometry. These studies were carried out over the temperature range 1073-1123 K. Boron-containing electroactive species is shown to reduce quasi-reversibly at low scan rates (v < 0.1 Vs-1) and irreversibly at higher scan rates (> 0.1 V s-1) through a single-step three-electron process (B(III) + 3e → B). The transfer and diffusion coefficients of the electroactive species was measured for sodium fluoroborate in KCl-KF melt over the temperature range 1073-1123 K.
Keywords: Sodium fluoroborate; Cyclic voltammetry; Chronoamperometry; Diffusion coefficient; Melt.
Introduction
Boron carbide containing boron enriched in 10B isotope (67 at.%) will be used in the control rods of the Indian Prototype Fast Breeder Reactor (PFBR) [1]. An indigenous process developed for fabricating high-density boron carbide pellets for this application envisages the synthesis of boron carbide from enriched boron by reacting it with carbon [2]. A high temperature (1073 K) molten salt electrolysis process was developed for the synthesis of high purity boron [3 -5] suitable for control rod application in fast reactors. The electrolyte employed is a melt comprising KCl (72.96 mol %), KBF4 (11.04 mol %) and KF (16 mol %) [3]. In order to have a more fundamental insight into the process with different melt compositions, a detailed experimental programme is currently being pursued at the Indira Gandhi Centre for Atomic Research (IGCAR), Kalpakkam, India. The electrochemistry of KCl-KF-KBF4 (1.6 × 10-4 to 7.73 × 10-4) melts has been reported in our previous study [5]. A systematic study on boron deposition from other alkali metal fluoroborates in KCl-KF melt has not been reported yet. The electrochemistry of boron is being studied since 1925 due to its nuclear and non-nuclear industrial applications [6-9]. Researchers have studied electrodeposition of elemental boron from various boron containing melts [1022]. In this context fluoroborate containing melts have been extensively studied [23-30]. The reduction of B(III) in NaCl-KCl-MBF4 (M = Na and K) melts was studied by Taranenko et al. [25]. The concentration of MBF4 in these melts varied from 3 × 10-5 to 8 × 10-4 mol cm-3. The voltammograms were recorded on a glassy carbon electrode at 973 K. The reduction potential of B from B(III), with respect to an Ag/AgCl reference electrode, involving a single-step three-electron transfer process, was reported by these authors. The decrease in concentration of the electrochemically active species in the melt with time was reported to be due to chemical reaction of tetrafluoroborate with the chloride melt and (or) by the thermal decomposition of tetrafluoroborate. According to Danek et al. [31], the dissolution of KBF4 in MCl (M= Li, Na, K) melts was proposed to follow the following exchange reactions:

The KBCl4 thus formed decomposes according to Eqn. 2.

The stability of BF4-anions in molten alkali chlorides was reported to increase as LiCl < NaCl < KCl due to the strong polarizing effect of the cation (Li+ > Na+ > K+) [25]. Decomposition of BCl4-ions was not observed in NaCl and KCl melt system. It was assumed that MBF4 thermally decomposed to gaseous BF3 according to Eqn. 3.

Thermodynamic analysis substantiated that the addition of F -ions to the chloride melts shifted the equilibrium of Eqns. 1 and 3 to the left and consequently suppressed the decomposition of the electrochemically active species. Thus chloride-fluoride supporting electrolyte was better suited compared to pure chloride melts for the electro-deposition of boron.
The aim of the present work is to investigate the electrochemical reduction of boron containing electroactive species in KCl-KF-NaBF4 melt and evaluate the kinetic parameters of the electro reduction process. Transient electrochemical techniques such as cyclic voltammetry and chronoamperometry have been used in the present study.
Experimental
Materials
KCl (99.5 % purity) and KF (99 % purity) were supplied by M/s. Loba Chemie Pvt. Ltd., Mumbai, India. NaBF4 (> 98% purity) was obtained from M/s. Sigma Aldrich, USA. High-density (1.85 g cm-3) graphite was procured from M/s. Pure Components Pvt. Ltd., Pondicherry, India. Platinum wire (1 mm dia., 99.99% purity) was procured from M/s. Sevantilal Mahasukhlal, Mumbai, India. Inconel 600 (99.9% purity) which formed the structural material of the electrochemical cell was supplied by M/s. Kalapurna Engineering, Mumbai, India.
Electrochemical studies
Voltammetric studies were carried out over the temperature range 1073-1123 K. The electrochemical cell described elsewhere [5] was operated under argon during the measurement. The experiments were conducted using a three-electrode assembly. A high-density graphite crucible served as the container for the electrolyte as well as the auxiliary electrode. Platinum wire (1 mm diameter) served as the working electrode. The surface area of the Pt electrode dipped in the electrolyte was kept constant at 0.23 cm2 for each measurement. The potentials were measured against an Ag/AgCl reference electrode developed in our laboratory [32]. The voltammograms were measured using the electrochemical workstation Autolab (PGSTAT 302N) equipped with an IF 030 interface.
Chronoamperometric studies
Chronoamperometric measurements were carried out employing a platinum electrode (0.23 cm2 area) by applying potentials ranging from -0.88 to -0.85 V for a short duration of 5 s for the KCl (81.54 mol%)-KF (18.45 mol%) melt with NaBF4 (1.67 × 10-4 mol cm-3) over the temperature range 1073-1123 K. Chronoamperograms were also measured using Autolab (PGSTAT 302N) equipped with an IF 030 interface.
Analysis of boron in the melt
After the completion of the voltammetric scans, melt samples were withdrawn from the cell and analyzed. About 10 mg of the solidified melt were dissolved in 10 mL of 0.02 N HNO3. The solution was then analyzed for its boron content by inductively coupled plasma mass spectrometer (ICP-MS, Model: Elan 250, M/s. Sciex, Toronto, Canada) after suitable dilution. These measurements had a standard deviation of about 3 to 5% of the reported value.
Results and discussion
Cyclic voltammetry
The cyclic voltammograms (CV) of the melt KCl (81.54 mol%)-KF (18.45 mol%)-NaBF4 (1.67 × 10-4 mol cm-3) (designated as melt A) were recorded over the temperature range 1073-1123 K. Typical cyclic voltammograms of this melt at various scan rates (0.05 - 2.0 V s-1) are shown in Fig. 1.
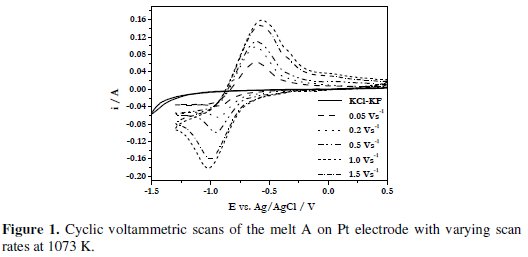
It is seen that the cathodic peak shifts to a more negative potential and the anodic peak shifts to a more positive potential with increasing the scan rates. In addition, the peak current increases with increase in the scan rate.
Fig. 2 shows the CVs of melt A at 1073, 1098 and 1123 K.
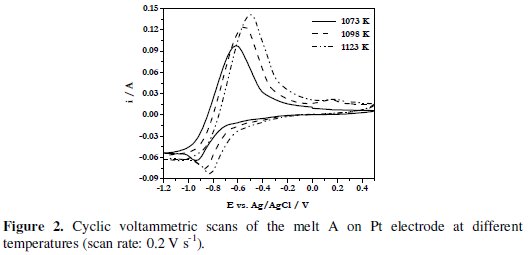
The cathodic (Epc) and anodic (Epa) peak potentials of the electroactive species as well as the average of cathodic and anodic peak potentials, (Epc + Epa)/2, of this melt at different temperatures are given in Table 1.
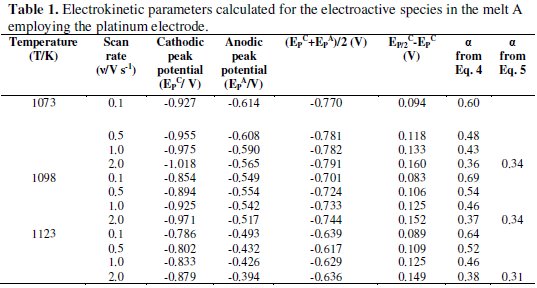
It is observed that the cathodic and anodic peak potentials are shifted to less negative values (Fig. 2) and the average values increase with increase in temperature (Table 1).
It is also observed that the magnitude of Ep/2c - Epc, (Ep/2c is cathodic half-peak potential), is larger than the value required for the reversible process of formation of an insoluble substance (0.071/n) × V at 1073 K where, n is the number of electrons transferred for the reduction process) [33, 34], indicating that the reduction of boron species on platinum electrode from KCl-KF-NaBF4 melt is not reversible.
The reduction potential of boron from boron containing electroactive species in the melt A was found to be more negative compared to that of melt KCl (81.54 mol%)-KF (18.45 mol%)-KBF4 (1.6 × 10-4 mol cm-3) (designated as melt B) [5] (Fig. 3).
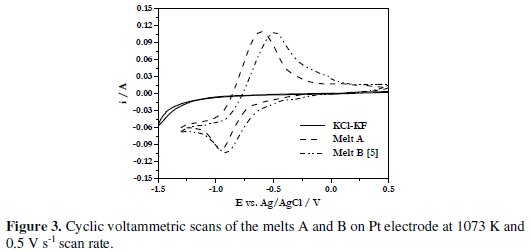
Fig. 4 shows the plot of ipc / v1/2 vs. v1/2 where, ipc is the cathodic peak current and v is the scan rate.
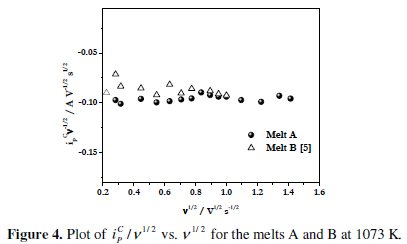
It is observed from these plots that for the melt A at 1073 K, the ipc / v1/2 values remain almost constant at polarization rate range of 0.05 to 2 V s-1. This suggests that the process of electrodeposition of boron on Pt electrode is not complicated by chemical reactions as suggested by Tsiklauri et al. [35, 36]. In addition, the electrodeposit is not adsorbed at the electrode surface. A similar behaviour was observed for the melts NaCl-KCl-KBF4 [24] and melt B [5]. Thus it can be inferred that the electrodeposition of boron from alkali metal fluoroborate containing KCl-KF melts is not complicated by chemical reactions.
The variation of Epc with logarithm of scan rate, log(v), at different temperatures for the melt A is shown in Fig. 5.
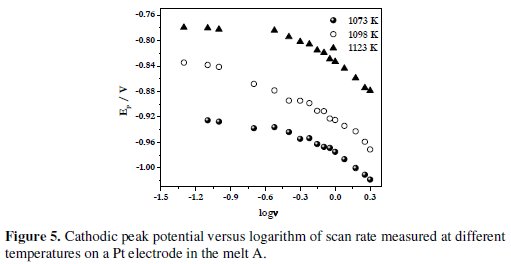
As seen from the figure, the cathodic peak potential varies linearly with log(v). These observations indicate that the reduction of electroactive boron species on platinum electrode is quasi-reversible at low scan rates (v < 0.1 Vs-1) and irreversible at higher scan rates (> 0.1 V s-1).
The variation of the cathodic peak current, ipc, with the square root of scan rate, v1/2, at different temperatures for the melt A is shown in Fig. 6.
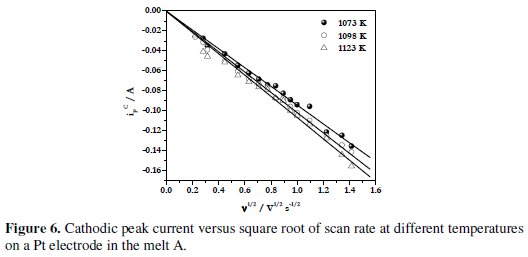
The linear relationship between ipc and v1/2, as observed from these figures indicates that the process is diffusion controlled.
Calculation of transfer coefficient
The value of αnα (where α is the transfer coefficient and nais the number of electrons transferred for the reduction process in the rate determining step) can be determined from the equation given by Matsuda and Ayabe (Eqn. 4) [37, 38].

where R is the molar gas constant, T is the absolute temperature and F is the Faraday constant.
αnα also can be evaluated from the following equation [33].

where ΔEpc/Δlog(v) is determined from the slope of Epc vs. log(v) plots.
The transfer coefficients calculated from Eqns. 4 and 5 for the melt A are given in Table 1. For the melt A at 1073, 1098 and 1123 K the transfer coefficients were found to vary from 0.60 - 0.36, 0.37 - 0.69 and 0.64 - 0.38, respectively. By using Eqn. 5 the transfer coefficients for the melt A were found to vary from 0.34 - 0.31. The typical transfer coefficient values signify that the process is irreversible. Similar inference has also been drawn for the melt B reported in our previous study [5].
Diffusion coefficients
The diffusion coefficient of the electroactive species can be measured by chronoamperometry using Cottrell equation (Eqn. 6) [33].

where A is the electrode area in cm2, C0* is the concentration of B(III) ion in mol cm-3, D0 is the diffusion coefficient in cm2 s-1, F is the Faraday constant, i is the current in amperes and t is the transition time in s. Typical chronoamperograms of the melt A employing Pt electrode are shown in Fig. 7.
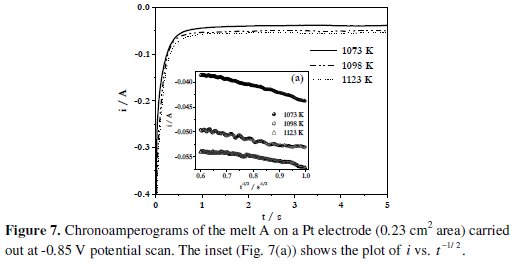
A linear variation of i with t-1/2 is observed as shown in Fig. 7(a). This indicates that the reduction of boron containing electroactive species in these melts is diffusion controlled. The diffusion coefficients of the electroactive species in the melt A were found to be 6.4 × 10-6 cm2 s-1 at 1073 K (Table 2).
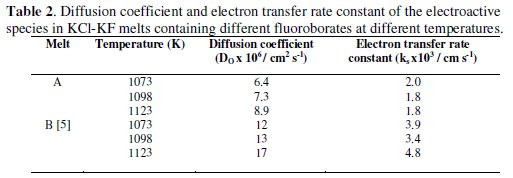
Typical diffusion coefficient values for different alkali metal fluoroborate containing melt system at different temperatures are also presented in Table 2.
A comparative study of D0 values of the boron containing electroactive species from different alkali metal fluoroborates in KCl-KF melt shows that D0NaBF4 < D0KBF4. According to Stokes-Einstein equation (Eqn. 7), the diffusion coefficient of a species is inversely proportional to its solvodynamic radius ( rs ).

where k is the Boltzmann constant, C is a constant and η is the absolute or bulk viscosity of the solvent. It is surmised that the solvodynamic radius ( rs ) of the boron containing electroactive species varies as rsNaBF4 > rsKBF4 in KCl-KF melt containing different alkali metal fluoroborates.
Electron transfer rate constant
Klingler and Kochi [39] derived an expression (Eqn. 8) for obtaining the electron transfer rate constant ( ks ) by assuming that the electron-transfer reaction is irreversible.

By using the above equation the electron transfer rate constants for the irreversible electrode process for sodium fluoroborate in KCl-KF melt over the temperature range 1073 to 1123 K were calculated. The ks values obtained by substituting the αnα values derived from Eqn. 4 at 1 V s-1 scan rate and DO from Eqn. 6 are presented in Table 2. These values indicate sluggish electron transfer kinetics due to the fact that the oxidized boron (fluoro-chloro complex of boron) and the reduced boron (elemental boron) are structurally dissimilar and the activation barrier for the redox process is large.
Apparent surface resistance
Polyakova et al. [34] reported that the broadening of the cathodic peak in CV occurred due to the ohmic control of deposition of boron. These authors presented linear plots of peak current and peak potentials against the square root of scan rate and concluded that a surface boron film formed on the electrode rendered the process to be controlled by ohmic resistance. The ''apparent surface resistance'' ( Rapp ) of the electrolyte in the pores of the boron film covering the electrode can be obtained from the reciprocal of the slope of peak current density ( jp ) vs. peak potential ( Ep ) plots as reported by Polyakova et al. [34]. The plots for cathodic peak current density vs. cathodic peak potential for the melt A show a linear behavior. The values of R calculated for different fluoroborates in KCl-KF melt at different temperatures are presented in Table 3.
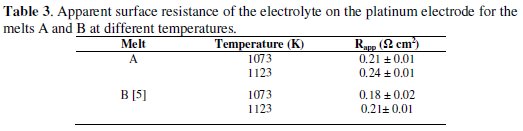
It is observed (Table 3) that the Rapp values decrease with increase in temperature. Polyakova et al. [34] reported a higher value of Rapp (3.3 Ω cm2 at 973 K) in the melt LiF-NaF-KF-KBF4 (15.9 × 10-2 mol%). In our earlier study on the melt B, the Rapp (0.18 ± 0.02 Ω cm2 at 1073 K) was found to be of similar magnitude [5]. Thus Rapp was found to be independent of the nature of alkali metal fluoroborate in KCl-KF melt. The typical resistance values obtained (Table 3) in our present study are very small and it is surmised that the effect of resistance on CVs is negligible and it does not quite affect the determination of α values from the cyclic voltammetric scans.
Conclusions
The electrochemical reduction of B(III) + 3e → B in KCl (81.54 mol%)-KF (18.45 mol%)-NaBF4 (1.67 × 10-4 mol cm-3) melt on a Pt electrode was studied by cyclic voltammetry over the temperature range 1073 to 1123 K. It is inferred that at a sweep rate of v < 0.1 Vs-1 the electroreduction is quasi-reversible and at v > 0.1 Vs-1, both the diffusion of electroactive species and the electron transfer play a role in the reduction process. It is established that the reduction of B(III) to B occurs by a single-step three-electron process. Using chronoamperometry, the diffusion coefficients of the boron containing electroactive species (B(III)) were calculated for the sodium fluoroborate melt system and it was found that D0NaBF4 < D0KBF4. The values of α and Rapp were found to be independent of the nature of alkali metal fluoroborate in KCl-KF melt.
References
1. Babu V R, Govindarajan S, Chetal S C. Proc of Seminar on Inherent Engineered Safety Aspects of PFBR Design. IGCAR: Kalpakkam, India; 1996. [ Links ]
2. Suri A K, Subramanian C, Sonber J K, et al. Int Mater Rev. 2010;55:4. [ Links ]
3. Jain A, Anthonysamy S, Ananthasivan K, et al. Mater Charact. 2008;59:890. [ Links ]
4. Pal R, Ananthasivan K, Anthonysamy S, et al. Electrochim Acta. 2012;61:165. [ Links ]
5. Pal R, Anthonysamy S, Ganesan V. J Electrochem Soc. 2012;159:F157. [ Links ]
6. Brotherton R J, Steinberg H. Progress in boron chemistry. Pergamon Press Inc; 1970. Vol 2. [ Links ]
7. Matkovich V I. Boron and refractory borides. Berlin: Springer Verlag; 1977. [ Links ]
8. Subramanian C, Suri A K. Metal Mater Processes. 2004;16:39. [ Links ]
9. Davidson M G, Hughes A K, Marder T B, et al. Contemporary Boron Chemistry, Royal Society of Chemistry, Cambridge 2000. [ Links ]
10. Kahlenberg H H. Trans Am Electrochem Soc. 1925;47:23. [ Links ]
11. Andrieux L. Ann Chem Phys. 1929;12:432. [ Links ]
12. Andrieux L, Deiss W. Bull Soc Chim. 1955;838. [ Links ]
13. Cooper H S. US Patent 2572248;1951. [ Links ]
14. Cooper H S. US Patent 2572249;1951. [ Links ]
15. Russel S, Young F A, Kellner J D. US Patent 3843497;1974. [ Links ]
16. Murphy N F, Tinsley R S. US Patent 2848396;1958. [ Links ]
17. Uchiyama A A, Stern D R, McKenna Q H. US Patent 2940911;1960. [ Links ]
18. Stern D R, McKenna Q H. US Patent 2892762;1959. [ Links ]
19. Ellis R B. US Patent 2810683;1957. [ Links ]
20. Nies N P, Fajans E W, Thomas L L, et al. US Patent. 2832730;1958. [ Links ]
21. Nair K U, Bose D K, Gupta C K. Miner Process Extractive Metall Rev. 1992;9:283. [ Links ]
22. Miller G T. J Electrochem Soc. 1959;106:815. [ Links ]
23. Brookes H C, Gibson P S, Hills G J, et al. Trans Inst Met Finish. 1976;54:191. [ Links ]
24. Kuznetsov S A. Russ J Electrochem. 1996;32:763. [ Links ]
25. Taranenko V I, Zarutskii I V, Shapoval V I, et al. Electrochim Acta. 1992;37:263. [ Links ]
26. Kellner J D. J Electrochem Soc. 1973;120:713. [ Links ]
27. Makyta M, Matiasovsky K, Fellner P. Electrochim Acta. 1984;29:1653. [ Links ]
28. Akashi K, Egami I. Seisan-Kenkyu. 1963;15:427. [ Links ]
29. Newkirk A E. Elemental boron. In: Adams RM, editor. Metallo-boron compounds and boranes. New York. Interscience Publishers; 1964. pp 301371.
30. Kenkyujo S. Japanese Patent. 6243; 1966. [ Links ]
31. Danek V, Votava I, Matiasovsky K. Chem Zvesti. 1976;30:377. [ Links ]
32. Pal R, Ananthasivan K, Anthonysamy S, et al. Electrochim Acta. 2011;56:4276. [ Links ]
33. Bard A J, Faulkner L R. Electrochemical methods: fundamentals and applications. Wiley, New York; 1980. [ Links ]
34. Polyakova L P, Bukatova G A, Polyakov E G, et al. J Electrochem Soc. 1996;143:3178. [ Links ]
35. Tsiklauri O G, Shapoval V I, Avaliani Ash. Ionic Melts and Solid Electrolytes. 1986;1:47. [ Links ]
36. Tsiklauri O G, Avaliani ASh, Bairammashvili I A. Soobshch. Acad Nauk Gruz SSR. 1989;134:133. [ Links ]
37. Matsuda H, Ayabe Y. Z Electrochem. 1955;59:494. [ Links ]
38. Golovin V A, Dobrenkov G A. Trans Kazan Khim-Tekhnol Inst. 1965;34:202. [ Links ]
39. Klingler R J, Kochi J K. J Phys Chem. 1981;85:1731. [ Links ]
Acknowledgements
The authors are grateful to Mr. R. K. Prabhu for carrying out the ICP-MS analysis.
*Corresponding author. E-mail address: rahul.pal@curaj.ac.in
Received 10 May 2014; accepted 2 March 2015