Introduction
Neuroimaging in children is challenging and needs to be approached differently from adult imaging, due to anatomic specificities as well as rapid changes in structure, metabolism, and function of the paediatric brain.1
To obtain the highest possible quality images in this age group, paediatric radiologists/neuroradiologists must choose the most suitable method, equipment1 and technique to augment children compliance and imaging diagnostic accuracy, simultaneously avoiding any deleterious effects as much as possible.
Computed tomography (CT) is a fast, easily accessible, and widely used diagnostic tool. However, being an ionising radiation-based method, its use in the paediatric population leads to concerns regarding radiation-induced side effects, the most important of which related to the development of cancer,2 such as leukaemia or brain tumours.3 Other techniques, such as conventional radiography and fluoroscopy, also share the same concerns regarding x-ray exposure. To accurately ascertain ionising radiation exposure, it is crucial that neuroradiologists understand the effects and learn how to accurately estimate radiation exposure dosage to reduce radiation impact and avoid possible side effects.
Magnetic resonance imaging (MRI) is currently regarded as the most accurate imaging method for the evaluation of the brain and spinal cord, with the additional advantage of not using ionising radiation. Currently, the main disadvantage of MRI is still the long acquisition time, especially challenging in the paediatric setting, increasing the risk of motion artifacts and the need for sedation or general anaesthesia, increasing the cost and the imaging time and also posing potential additional risks to the patients. To overcome these drawbacks, new imaging techniques, adjusted devices and fast sequences have been developed and optimised. MRI examinations raise other concerns, namely biological effects of non-ionising electromagnetic fields (EMF), risks regarding noise exposure and hazards from ferromagnetic devices that need to be considered by the paediatric radiologist/neuroradiologist.
In specific contexts, there may be the need to use contrast media on either CT or MR, which poses additional potential risks, including allergic or renal secondary effects.
In this review we aim to describe the most used neuroimaging methods in the paediatric population, specific concerns and challenges that arise in this age group, aiming to provide safety recommendations and an action plan to optimise neuroimaging in the paediatric population.
A. Imaging Techniques
1. Ultrasonography
Paediatric head ultrasonography (USG) is a safe neuroimaging method, being both inexpensive, radiation-free, widely available and with the possibility to be performed at bedside, without major risks.4 As it can only be performed with fontanelle patency it is limited to specific age groups, but when fontanelles are not closed, USG can be a good imaging method to study acute intracranial pathology namely evaluating intracranial bleeding, oedema or major malformations. This imaging technique is also operator and equipment dependent, augmenting the rate of imaging variability.
Image gently is a coalition of healthcare organisations dedicated to providing safe, high quality paediatric imaging worldwide, founded by the Society for Pediatric Radiology, the American Association of Physicists in Medicine, the American College of Radiology and the American Society of Radiologic Technologists who emitted Paediatric CT Protocols.
This organisation provides some advice regarding USG,5 namely using USG as a strong resource to lower radiation dosage in children´s imaging, recommending the use of USG whenever possible.
Postnatal screening with USG is indicated for newborns at risk or with suspected brain injury. Risk factors and clinical signs of neonatal brain injury are present in table 1, according to Jeroen Dudink et al.6
2.1 CT scan
The use of CT imaging has risen steeply in recent years, being the most used diagnostic method in the overall population, especially in trauma settings, providing very fast acquisition of information about the brain, skull and spine. However, this imaging method is less useful to study the brain parenchyma, the cranial nerves, and the meninges or the spinal cord, namely for neoplastic, infectious, or inflammatory conditions.
A major drawback of CT is the use of ionising radiation. Concerns on whether radiation exposure can lead to an increased risk of developing brain tumours in the paediatric population has emerged.2 The risk of ionising radiation cancer induction in children is considered to be approximately 10 times greater than in adults,4 relating to the growing of organs, with rapidly dividing cells, that may be more susceptible to radiation but also, related to the children’s longer expected life with more time to manifest possible long term alterations.4,7The overall cancer incidence for children who have undergone one or more CT scans has been described to be 1.5 times higher than expected and this risk is estimated to increase linearly.3
For these reasons, precaution is recommended when using ionising radiation imaging methods in children, avoiding repeated or inappropriate examinations and, whenever needed, ensure that these are performed with the highest image quality and the lowest possible radiation dose, the “as low as reasonably achievable” (ALARA) concept.7
The American College of Radiology (ACR) recommends physicians to follow appropriateness criteria to determine if a CT scan should be performed in the paediatric population (Table 2, Table 3, Table 4) or if other modalities must be considered (MRI or sonography).8,9,10
Table 4: ACR appropriateness criteria for performing a head and neck CT scan in paediatric patient.10
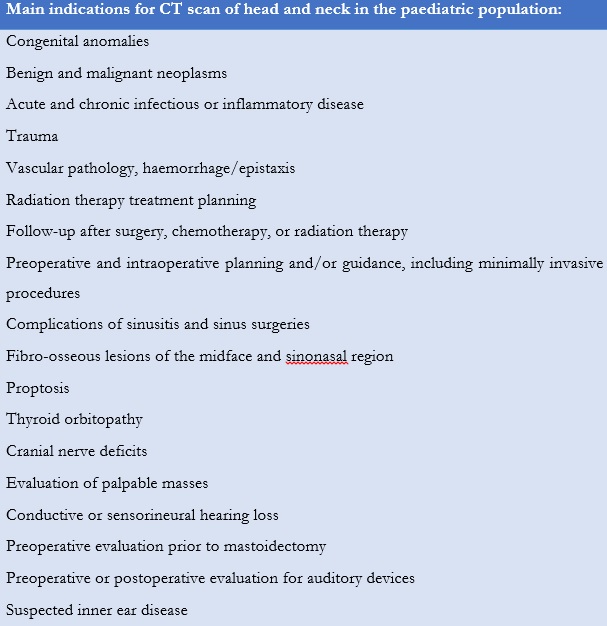
As the Image gently11 highlights, it is important to note that radiation reduction may be hard to achieve since it can increase the quantum mottle or background noise of CT images. Neuroradiologists should work closely with medical physicists to achieve the best equilibrium of lowest radiation dose and image quality for accurate diagnosis.11 Additionally, differences in CT scanner design can make it impossible to estimate patient radiation dose based on technique factors alone.11
The European Guidelines on Diagnostic Reference Levels for Paediatric Imaging12 were also created to highlight the importance of establishing diagnostic reference levels (DRLs) (a specified radiation dose for a given imaging study that is not expected to be exceeded) for medical examinations, in particular CT, radiography, and fluoroscopy, of patients sensitive to radiation, especially children.
Both volume computed tomography dose index (CTDIvol) (a standardised measure of radiation dose output measured in mGy) and dose length product (DLP) (a measure of CT tube radiation output/exposure measured in mGy*cm) are recommended quantities for setting DRLs, being relevant parameters for the patient dose burden per slice and patient dose burden for the complete CT procedure. To establish paediatric DRLs it is crucial to include the patient’s age, weight, required dose quantities, technical equipment parameters; these data must also be available for export enabling further analysis. The EDRLS for paediatric head CTs are presented in Table 5.
Table 5: EDRLS provided by The European Guidelines on Diagnostic Reference Levels for Paediatric for head CT (16 cm phantom CT in a complete routine CT examination.).12
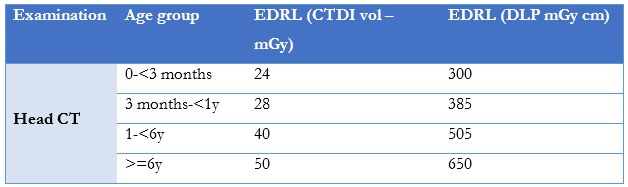
On the other hand, it is crucial to optimise patient positioning and to scan only the specific area of interest (“child-size” the scan),13 for example, trying to avoid the orbits (minimise radiation dose to the lens/prevent radiation-induced cataracts) in a brain CT-scan.10Additionally, protocols should include adjustments in the scan parameters including: 4,8
- Decrease rotation time (no more than 2 seconds
- Reduce field of view
- Reduce kilovolts
- Use of smart milliamperes/auto milliampere options
- Reduce table pitch to no greater than 2
- Limiting spatial resolution: resolution should be >10 lp/cm for a display field of view <24 cm (must be measured to verify that it meets the unit manufacturer’s specifications)
- Adjust slice thickness: acquired slice thickness should be 2 mm or less, whereas reconstructed slice thickness should be 5 mm or less
The American Association of Physicists in Medicine14 summed up the advantages and disadvantages in using either axial or helical scans for routine head CT and this decision must be influenced by specific patient indications, scanner availability and image quality requirements.14 Axial scans can have less artifacts and better image quality in some cases although radiation dose depends more on the protocol than on the mode of acquisition.
For paediatric head CT, ACR suggests axial scan with a slice thickness of no more than 5 mm, and reformatted images in coronal, sagittal and true axial, with at least two different windows, like brain/soft tissue and bone.8
2.2 Conventional Radiography
Image gently provides some advice regarding the usage of conventional radiography,15 namely the importance of measuring patient thickness to provide a “child-size” technique, avoiding grids for body parts less than 10-12 cm thick, usage of x-ray only in the indicated area with proper collimation and shielding and always checking exposure indicators and image quality.
According to The European Guidelines on Diagnostic Reference Levels for Paediatric Imaging,12 air kerma-area product (Pka) (which provides an indication of the radiation dose received by the patient, is calculated as the product of dose and beam area Gy.cm2) is the recommended primary DRL quantity for radiography and interventional radiology (IR), considering the full radiation exposure of the patient.12 In Table 6 we provide the European DRLS (EDRLS) of paediatric conventional radiography.
Table 6: EDRLS provided in The European Guidelines on Diagnostic Reference Levels for Paediatric of radiography.12
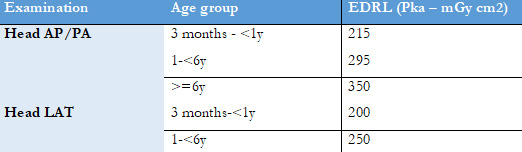
2.3 Angiography
The use of angiography in interventional radiology (IR) also has challenges in the paediatric population. Image gently elaborated the Steps for Radiation Safety in Paediatric Interventional Radiology16 with plan, prepare, communicate, measure, record and review as the main words to remember. In addition, issues related to a reduced contrast volume, injection rates, and radiation dose minimisation must be considered. In this regard it is very important to have a qualified physicist for equipment maintenance and quality assurance.
Both maximum radiation output and routine radiation output must be measured and documented, appropriate lengths of runs and different frame rates for slow versus high flow according to patient size should be considered and “child-size” protocols using dose reduction and low mA as possible should be used. In addition, dose recording and reduction technologies should be installed in the equipment and caregivers should be asked about previous radiation exposure and answer questions about radiation safety before the procedure.
During the procedure, appropriate communication is key, such as putting into action the previous planning (number of runs, injection, contrast and pump parameters, digital subtraction angiography (DSA) frame rates, lower the number of exposures, view and save images with last image hold), optimising patient position timing with anaesthesia, minimise live fluoroscopy (tap on pedal and examine the still image on monitor), use low pulse fluoroscopy (7 or 3/sec), collimate tightly, minimising use of electronic magnification and, if possible, move table away from x-ray tube in both planes to avoid radiosensitive areas. Room temperature must also be considered, usually at 18°-22°C, using blankets or warmers, particularly in neonates who are more vulnerable to develop hypothermia.4
After the procedure, a review and record of the radiation dose, including cumulative air kerma, is mandatory. If the indices are not available, recorded fluoroscopy time and estimations of the total number of recorded images can be used to estimate patient doses. It is important to remember that scattered radiation in the room is directly proportional to the patient dose, so reducing patient dose reduces scatter and dose to personnel also, since these are tied together.
For IR, no EDRL can be proposed because neither official nor suggested DRLs exist. Further research and data collection are needed to provide a reliable basis for suggesting DRLs.12
3. MRI
Magnetic resonance imaging (MRI) is a non-invasive and overall safe method to examine the human brain across the entire lifespan, including in the research setting. Compared to CT or x-ray, MRI does not use ionising radiation and has excellent resolution and tissue contrast.17 However, a few concerns regarding this imaging method remain that need to be considered. Indeed, the biological effects of non-ionising EMF as well as static magnetic fields, gradient fields and radiofrequency can cause tissue polarisation, inducing current and thermal heating2,18nevertheless, so far, the World Health Organization (WHO) has stated that the carcinogenicity of static magnetic fields to humans is not at present classifiable, and the FDA has approved exposure to neonates up to 4T fields.3
Despite this fact, caution should be taken regarding the children’s clothes/diapers, and metallic material, to prevent a skin rash or burn, and a rigorous process of screening for external and internal metal implants and metallic foreign bodies should be applied.19 Also, a protocol for performing MRI in children with implanted devices such as nerve stimulators and cardiac electronic devices, must also be established in conjunction with the cardiology or electrophysiology service or device vendors.19
On the other hand, the acoustic noise can rise to 132 dB,17 with a risk of hearing loss and neurosensory adverse effects.3 Therefore, earplugs should always be used, even in anesthetised patients.
Finally, the risk of ferromagnetic projectiles unintentionally carried inside the scanning room is greater in children with potentially serious risks.3 Indeed, children often need supplementary live supporting equipment, so having MRI compatible with these materials is fundamental. Regarding implanted devices, these should have terms to label MRI compatibility (in their absence, the manufacturer should be consulted), since they can determine artifacts, heating, or electromagnetic field interactions.
A typical MRI scan acquisition time varies normally between 15 to 60 minutes, depending on the sequences used, making it very sensitive to motion, which is a major challenge in children. Indeed, motion is a very prevalent MRI artifact that results in non-diagnostic examinations, especially in the paediatric population. There is an increased need to develop MRI techniques to reduce motion artifacts and the use of sedation/anaesthesia to perform the examinations, which are explored in a dedicated section below.20
B. Contrast media in paediatric neuroimaging methods
1. Iodinated contrast
Iodinated intravenous (IV) contrast media is used for paediatric CT studies but there are relatively few published guidelines on the respective appropriate administration.21
The reported incidence of paediatric allergic-like reactions to contrast is variable, but it is generally agreed that the incidence is lower than in adults.22 General guidelines to prevent or treat allergic-like reactions are like those used for adults, with adjusted dosages.22
Also, the effects of contrast on renal function are generally assumed to be similar between adults and children. Risk factors for contrast-induced nephrotoxicity are thought to be like those in adults and strategies described in adults should be considered.22
Iodinated contrast osmolality is crucial to consider; administration of hyperosmolar contrast may result in migration of fluid from the extravascular soft tissues into blood vessels, expanding blood volume, since children are highly susceptible to fluid shifts.22
Contrast viscosity is another important property to consider, as viscosity increases the pressure associated with an intravascular injection, an important factor due to the use of small gauge catheters in small blood vessels.22
Very small volumes of contrast are administered to children - typically 1.5-2mL/kg. Consequently, it is fundamental to consider the timing of contrast administration when performing certain studies, such as CT angiography; slower injection rates may be useful to prolong intravascular enhancement.22 Small-gauge catheters located in small peripheral veins are commonly used and, according to ACR22 the following associations are suggested:
Extravasation can also occur in children (reported rate of 0.3%) but in most cases resolves without sequels.22
It is important to always register contrast specifications, such as brand name, route of administration, and administered dose of the contrast agent for each examination.
Emergency equipment and medications must be always accessible if contrast media adverse reactions occur.
When iodine-based contrast agents (IBCA) are used in CT examinations there are additional radiation dose concerns. Iodinated tissues were found to receive higher radiation doses and the dose increases for tissues with high iodine uptake23 with the presence of IBCA increasing the radiation dose to various extents in different organs.24 X rays interact strongly with iodine since it is an inert element, like metal, influencing the radiation dose to DNA targets. It has been suggested that iodine may increase cancer risks.25Harbron et al.25 suggested that the impact of IBCA on cells outside the blood vessels, is likely overestimated, however, the dose to the blood vessels endothelium and to the heart may be underestimated which may have significant consequences.
It is possible to increase the CT tube current to accommodate the additional attenuation of the contrast agent24 and CT dosimetry of contrast enhanced CT should consider tissue iodine uptake.23
So, the usage of IBCA must be weighed very carefully in the paediatric population considering the associated risks of these contrast agents.
2. Gadolinium based contrast agents
Gadolinium-based contrast agents (GBCA) usage in children of all ages should be carefully assessed and the benefit of administration should outweigh potential risks.
Common indications for contrast use in children include infection, demyelination disorders, tumours, CSF and cranial nerve pathologies, neurocutaneous syndromes, and vascular pathologies.26
As with iodinated contrast, there is a significant range in osmolality and viscosity of GBCA, however these properties are less important when compared to iodinated contrast due to the smaller volumes that are used and the slower injection rates.22 Extravasation events are rare (reported rate of 0.05%).22
Dosage is not based on age, but weight-dependent (0.1 - 0.2mmol/kg body weight).26
When administered at clinical doses, the reported adverse events rate ranges from 0.07 to 2.4% in this age group.22
Though rare, allergic-like reactions do occur; mild reactions are more common and are treated similarly to those reactions to iodinated contrast.22 Premedication can be used, if necessary, especially in patients that had a prior reaction (these patients have about eight times a higher risk).22
A recent paper27 concluded that the risk of nephrogenic systemic fibrosis (NSF), using group II GBCAs in patients with advanced kidney disease is thought to be very low (0 events following 4931 administrations to patients with estimated glomerular filtration rate < 30 mL/min per 1.73 m2) and that dialysis initiation or alteration is likely unnecessary based on group II GBCAs administration. Depending on the clinical indication, the potential harms of delaying or withholding GBCAs for an MRI in a patient with acute kidney injury or eGFR less than 30 mL/min per 1.73 m2 should be balanced against and may outweigh the risk of NSF.27
The clinical significance and potential long-term consequences of GBCA deposition in the brain remain unclear and are important when there are repeated exposures.28However, macrocyclic agents, like gadobutrol, have controlled concentrations and, in current state of the art, are considered a safe and efficient MRI contrast agent to be used in the paediatric population.26
C. Sedation/general anaesthesia in paediatric neuroimaging studies
Chloral hydrate is the most frequently used sedative for children’s imaging, as it is readily available, easily administered, rapidly absorbed and has a relatively wide margin of safety with rare serious adverse events.29 Nevertheless, as previously mentioned, when anaesthesia is required, healthcare resources and expenses are increased30,31and imaging times are inevitably longer.30
In addition, under or over sedation may occur, leading to moving artifacts and decreased imaging quality, or respiratory problems such as desaturation or apnoea, respectively. Therefore, when general anaesthesia is needed, children undergoing MRI should be monitored, including electrocardiogram, blood pressure measure, pulse oximetry and continuous end-tidal carbon dioxide.32
Although some concern has been raised about the effect of anaesthetic exposure to the developing brain in recent years32 recent multi-national multi-institutional randomised controlled trial with follow-up at 2 and 5 years has shown that the use of single and short (i.e., less than 1 h) exposure of sedation/general anaesthesia in this young paediatric cohort is safe.33,34
To avoid sedation/general anaesthesia, some techniques should be attempted, including "Feed and wrap” in neonates and infants. In this method, food, comfort, and warmth are used to induce natural sleep and to reduce motion artifacts. Children must be fed 30-45 minutes prior to the scheduled scan time. Pacifiers and sucrose can be successfully used in neonates and infants younger than 3 months.32 If contrast administration is needed, an intravenous line should be placed prior to feeding. After feeding, the baby must be put to sleep in a quiet room with dim lights. Then, blankets are wrapped around the children's body to assure positioning as well as for noise protection.35 Sleep deprivation and melatonin hormones before imaging are other techniques that may improve the collaboration and success of MRI, with or without sedation.32 This technique should be tried at least once for non-emergent MRI in most infants ≤6 months to obviate the need of anaesthesia and the risks that come with it and increased consumption of healthcare resources.30 Approximately 75% of infants aged under 3 months can be scanned successfully for 45 min.36,37Possible hazards include overheating and consequences of over swaddling such as respiratory compromise, so heart rate and oxygen saturation must be monitored throughout the scan.35,36If children are awake and move, refeed must be tried until asleep again and the scan may be reattempted.
In addition, when sedation or anaesthesia is required, it may be used as an opportunity to perform certain additional invasive procedures.19
Use of fast MRI brain sequences is another possible strategy to avoid MR sedation in selected cases. These sequences have a very short acquisition time, usually less than 1 minute. The most common techniques are Half-Fourier Acquisition Single-shot Turbo Spin Echo (HASTE), Single Shot Fast Spin Echo (SSFSE), and Periodically Rotated Overlapping Parallel lines with enhanced reconstruction (PROP) FSE (Fast Spin Echo).38 However, the poor contrast resolution inherent to these protocols must be kept in mind in order to avoid potential pitfalls.
Standard sequences are normally used and, as in uncooperative adults, sequences may be prioritised in order of clinical importance.39
It is also preferable to run louder sequences such as diffusion-weighted imaging using echoplanar imaging at the end to minimise waking an infant if using the feed-and- swaddling non-sedate technique.39
On MRI, it is also possible to apply motion correction during or after image acquisition. A few existing methods include prospective motion correction updating the position of the field of view in real time dependent on motion estimates, as well as retrospective techniques using mathematical properties of the Fourrier transform, compressed sensing or machine learning.40 Radial k-space sampling techniques and variations, like two-dimensional radial T2- weighted sequences (PROPELLER - periodically rotated overlapping parallel lines with enhanced reconstruction) can also help decrease respiratory motion artifacts.39
Additionally, currently, there are deep learning-based reconstruction MRI techniques used in synthetic MRI (a multiecho sequence that simultaneously provides quantitative MR imaging and multiple contrast-weighted images in a single scan that can reduce scan time by 42% while maintaining image quality, lesion detectability and providing consistent quantitative values, that can be of great value in paediatric neuroimaging.41
Regardless of the imaging technique, distribution of informative booklets and educational videos with the most frequently asked questions, as well as divulgation of website addresses with virtual visits and simulation of the procedure36 are recommended in all suitable cases to reduce anxiety and augment the children's compliance. In addition, previous visits to the radiology department and interactions with small-scale MRI models also increase the chances of successful neuroimaging without the need of sedation. Finally, to try to create a less negative experience for the child, waiting rooms could be more child-friendly, like painted walls with colourful characters and have sufficient distractions.4,39Also, distraction strategies, such as listening to music, watch movies or use virtual reality devices considered for children, may decrease anxiety and improve the children’s experience during the examination.42
D. Recommendations
Based on this review we propose some recommendations for Paediatric neuroimaging (Table 7).
In paediatric imaging a multidisciplinary team approach with physicists, technicians, neuroradiologists, attending physicians and parents with appropriate communication is crucial. The individual risk versus benefit analysis should be performed in all cases when considering a neuroimaging examination in deciding whether to perform it and to select the best imaging technique according to the child characteristics and clinical information. After this decision is made, a detailed explanation of the procedure must be given to the children’s caregivers, as well as a review of all safety considerations.43
Finally, although an effort to avoid general anaesthesia in paediatric neuroimaging should be attempted, it is important to keep in mind that poor quality images or not performing the appropriate examination due to safety concerns can also have harmful effects on the patient’s care.
We also propose an algorithm of action based on individual risk/benefits assessment to provide neuroimaging safety in paediatric patients (Figure 1):
Conclusion
Neuroimaging methods are a crucial diagnostic tool in the paediatric population but pose distinct challenges from adult patients and possible secondary problems that need adequate specific answers. Awareness of paediatric safety is essential, and the selection of specific imaging techniques should be decided on a case-by-case basis, considering the specific age of the child, the clinical information, and the urgency of the examination, as well as its efficacy, usage of ionising radiation, availability, and cost.
A dedicated medical team and staff and available adequate imaging hardware and software are key features to succeed in paediatric neuroimaging, namely, to provide the best imaging data for both clinical and research purposes with the minimal risk profile.