INTRODUCTION
Hydrocarbon polluted soils are a widely extended contamination due to the intense use of petroleum derived products, petrochemical industries, and worldwide crude oil transportation, affecting even pristine areas of our planet. Thermal desorption and volatilization have been the most common treatments for heavily hydrocarbon polluted soils, but they are expensive and not exempt of environmental and health risks (Al Mutairi et al., 2008).
The application of bioremediation techniques for oil contaminated soils has been recognized as a cost-effective, very promising, and sustainable clean-up technologies (Wu et al., 2016). However, the drawback of these technologies is the short viability in soil of introduced microbial consortia, whose persistence in the soil is generally low. Microbial immobilization onto porous carriers could enhance their survival by means of biofilm formation in the inner spaces of the support. Biochar has been widely reported as a potential immobilization matrix, with the merits of high carbon content, cation exchange capacity (CEC), porosity, stability, and abundant surface functional group. In this way, biochars are used for water and soil remediation (Wu et al., 2022).
The aim of this work was to test the effectiveness of two biochar additions and the use of rhamnolipids to increase the degradation of recalcitrant hydrocarbons presents in an oil polluted soil.
MATERIAL AND METHODS
A soil polluted with heavy fractions of hydrocarbons and trace elements was collected from a machinery park. The soil has a sandy loam texture (11.8% clay, 29.8% silt, 58.3% sand), bulk density 1.51 kg L-1, highest water retention capacity (HWRC) 25.33%, pH (1:5; w:V) 7.1, Electrical conductivity (1:5; w:V) 0.839 dS m-1, Loss on Ignition 3.85 %, Organic Carbon 2.59%, Total N 0.02%, lime content 35.08%. Total trace elements concentrations (mg kg-1) were: As 77.3; Cd 7.8; Cr 14.9; Cu 8.5; Ni 9.9; Pb 339.2; Zn 680.5. Total petroleum hydrocarbons (TPHs) concentration is 4,272 mg kg-1.
Two biochar, produced from apricot stones pyrolyzed at 450°C (BH1) and 650°C (BH2), were used as microbial carriers at a rate of 5% (v/v); commercial rhamnolipids (RML, Sigma Aldrich) at 1% (w/v) were also assayed to increase hydrocarbon mobility. A microbial consortium previously isolated from this soil was grown in a minimal medium using diesel (1% v/v) as a sole C source. A concentrated suspension of this consortium in modified Bushnell Haas Broth (mBHB) was immobilized onto both biochars or rhamnolipids and mixed with the soil to reach a final volume corresponding to 40% HWRC.
Eight incubation treatments were prepared in 200 g soil microcosms (Table 1) for a 90-days incubation at 22°C, with periodic control of aeration and humidity. A second consortia inoculation (day 43rd) was performed increasing soil humidity to 50% of HWRC by adding the calculated amount of mBHB solution with inoculum or Milli-Q water. The nutrient solution (mBHB) had the following composition (g kg-1 dried soil): NH4NO3 3.543; K2HPO4 0.306; KH2PO4 0.306; MgSO4 0.051; CaCl2 0.006; FeCl3 0.015 (added separately). Microcosms were destructively sampled at 2, 15, 30, 45, 60 and 90 days.
Analysis of extractable petroleum hydrocarbons (EPH), basal respirometry, and the chemical properties were performed according to standard analytical methods. One-way ANOVA and LSD post-hoc test were applied to seek for differences between treatments.
Table 1 Incubation treatments
Samples | Treatment |
---|---|
4CT | Soil + water |
BH1 | Soil + BH1 + water |
BH2 | Soil + BH2 + water |
RML | Soil + rhamnolipids + water |
BA4CT | Soil + consortium + mBHB |
BABH1 | Soil + immobilized consortium-BH1 + mBHB |
BABH2 | Soil + immobilized consortium-BH2 + mBHB |
BARML | Soil + consortium + RML + mBHB |
RESULTS AND DISCUSSION
Different mechanisms are involved in the remediation of hydrocarbon polluted soils: volatization of the lighter molecular weight fractions, degradation by autochthonous microorganisms, or immobilization by humic substances or clays. This polluted soil was stored for a period higher than two years and the most volatile and degradable of EPHs had disappeared by natural attenuation before to star our bioremediation process. Initial characterization of EPHs showed that 82% of these compounds corresponded to long-chain hydrocarbons, with a molecular weight in the range C22-35, which supposes an important challenge for their biodegradation, due to their low mobility and availability.
Results obtained after 90-days of incubation under optimal conditions of humidity and temperature (Figure 1), showed the high recalcitrancy of these EPHs to microbial degradation, in which only the introduction of biochar, mainly with BH2, slightly reduced (around 10%) the total content of EPHs. On the contrary, bioaugmentation treatments displayed a higher hydrocarbon degradation capacity by the action of the added microbial consortium alone (BA4CT, 23%) or after immobilization onto biochars (BABH1 and 2) or with the addition of rhamnolipids as biosurfactants (BARML), all of them with yields close to 30% of initial EPHs’ concentration.
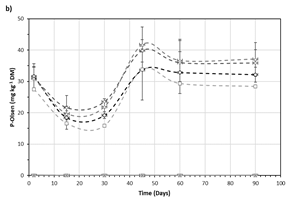
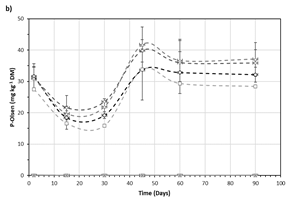
Figure 2 - Evolution of extractable total N (a) and available P (b) concentrations in the different treatments during 90 days of incubation.
The characteristics of the soil, with a scarce presence of soluble or available nutrients such as N and P, supposed an important constrain to soil biological activity.
The addition of mBHB medium in the suspended consortium supposed an important increase in extractable nitrogen (Figure 2a) or available P (Figure 2b), exceeding the nutrient demand of the added consortium.
Bioremediation of hydrocarbon polluted soils could be efficiently achieved through bioaugmentation, which involves the soil inoculation and enrichment with a specific consortium of microorganisms with high degradation capability (Yu et al., 2005).
In this work, the microbial consortium was previously isolated from this polluted soil and its microbiome was dominated by bacterial species of genus Pseudomonas, Achromobacter, Cupriavidus, Comamonadaceae and Sphingomonadaceae. Metagenomic data identified genes encoding enzymes implicated in the initial oxidation of alkanes: Alkane 1-monooxygenase (AlkB), long-chain alkane monooxygenase (LadA), cytochrome P450 alkane hydrolase (CYP153 family) and a variety of hydroxylating and ring-cleavage dioxygenases, involved in aromatic and polyaromatic hydrocarbon degradation that assured an efficient degradation of complex mixtures (Garrido-Sanz et al., 2019).
Our results clearly showed the viability of this consortium as demonstrated by the enhanced basal respiration of the soil mixture after microbial addition (Figure 3). However, this increased microbial activity was not enough to degrade high molecular weight aliphatic and aromatic hydrocarbons. The reduced bioaccesibility of these compounds to the added microorganisms, is a key limiting factor in the bioremediation of an aged and polluted soil.
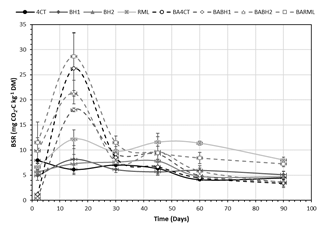
Figure 3 Evolution of basal soil respiration (BSR) in the different treatments during 90 days of incubation.
Under these conditions, the immobilization of the inoculum does not represent a clear advantage to increase the degradative pathways of hydrocarbons in this soil, such it occurs in liquid media amended with diesel. Also, a possible negative effect of biochar is perceptible in the form of inhibited respiration at the beginning of the incubation, mainly with the immobization in BH1, the biochar obtained at lower temperature, that usually drives to more functional and reactive surfaces, instead of most carbonaceous matrix that characterizes the products obtained at high pyrolysis temperatures.
CONCLUSIONS
The introduction of an immobilized microbial consortium increased the biological activity in the soil, but only a limited bioaccessibility improvement to the targeted contaminants was achieved.
Current research must be focused on the use of chemicals such as oxidants or stronger surfactants, that improve the mobility of heavy fractions of hydrocarbons.