INTRODUCTION
Triathlon is one of the most flourishing sports in the world. The only to mix three different sports (swimming, cycling and running) with different races and distances: short distance (750m, 20km, and 5km); Olympic distance (1.5km, 40km, and 10km); half distance (1.9km, 90km, and 21.1km) and full distance (3.8km, 180km, and 42.2km)(Olcina et al., 2018).
Compared to individual sports, the great challenge in triathlon is integrating three distinct modalities that differ from the others in duration, exercise mode, limb movement patterns, and main muscular groups (Sleivert & Rowlands, 1996). The success in triathlon has been related to the specific performance of each sport (swimming, cycling, and running) and its technical and/or tactical approaches during the race(Ofoghi, Zeleznikow, Macmahon, Rehula, & Dwyer, 2016).
The triathlete level performance depends on several factors (anthropometry, physiology, biomechanics, nutrition, or environmental conditions) (Olcina, et al., 2018). As it is performed outdoors, environmental aspects also influence the effort perception and thermal load, affecting the performance during the race (Logan-Sprenger, 2019). The influence of the environment is, in general, a growing reflection, and it is often neglected even though environmental stress can have a considerable impact on performance and safety (Whyte, Loosemore, & Williams, 2015).
Effectively, among environmental factors, the amount of water vapor in the air (absolute humidity) generates a great influence because the pressure gradient of the water vapor between the skin and the environment leads to cooling through evaporation by sweating (Wenger, 1972). The temperature and air velocity (for example, relative wind) are other contributors to the total heat exchange, in addition to radiant heat (Pezzoli, Cristofori, Moncalero, Giacometto, & Boscolo, 2013). Consequently, the clothes used modify the heat exchange rate, which is a preponderant factor for cooling through perspiration (Brocherie, Girard, Pezzoli, & Millet, 2014).
More recently, total body mass and body surface were clearly identified as important independent factors on thermoregulated results during exercise (Cheuvront & Haymes, 2001; Neves, Salamunes, de Oliveira, & Stadnik, 2017). Long-distance runners showed higher evaporative heat loss when the ambient temperature increased. Consequently, neglecting the body composition led to an underestimation of the restricted evaporation stress. All these characteristics can lead to diseases caused by heat, such as heat exhaustion (Armstrong et al., 1996).
However, most triathletes own and wear new wearable technology during racing and training that allow the athletes to track time, distance, speed, pace, heart rate, power output, and many other variables. This technology could produce information on physical performance-related variables with physiological measurements during each of the three modalities of an actual race. This can improving ability to determine factors associated with race total time(- Buckingham, 2018).
Furthermore, endurance exercises increase cytokine responses and reactive oxygen species (ROS) production. In these cases, antioxidant defenses are mostly required to protect the body cells from oxidative damage (Mrakic-Sposta et al., 2020). These biochemical responses in endurance activities are important to understand the physical performance during a competition (Santos, Neves, Fortes, Martinez, & Júnior, 2018) and can serve as a basis for the development of training programs for the triathlon. From the material published on the triathlon modality, no study was found that involved a set of environmental, biochemical, and subjective variables in relation to the acute effects of triathlon competition. Thus, the aim of this study was to analyze the acute effects of the endurance triathlon race on physiological markers and subjective perception variables in athletes.
METHODS
Participants
Participants were selected among places of triathlon training in Rio de Janeiro city, and consisted of 8 male triathletes, age between 25 and 39 years-old, with race experience and a week training volume of six days per week and three hours per day.
To be included in the study, participants should: no apparent risk factors that could prevent their participation in the study according to the Risk Stratification Criteria of the American Heart Association - AHA(ACSM, 2014), trained male athletes who used to compete in standard (Olympic) and endurance (long-distance) at least one year; age between 25 and 39 years, who trained regularly and answered the Par-Q questionnaire negatively (ACSM, 2018).
The study adopted the following exclusion criteria: athletes who did not finish the race; who used some medicine or substance which could influence the blood sample; who lost one of the data collection moments; who presents pathology or muscle articular injuries, or others clinic scream that could influence the results and performance.
The sample size was estimated by the G Power 3.1 software. As input, were informed to Test family: F tests; Statistical test: ANOVA: repeated measurements, within factors, effect size = 0.5, α = 0.05, power (1-β err prob) = 0.95, number of groups = 1, number of measurements = 4 and correlation coefficient among repeated measures = 0.5. The G Power 3.1 estimated the need for a total of 10 individuals.
At the end of the sampling process, the study had 10 volunteers, but two athletes dropped out of the study due to personal reasons. Thus, the sample consisted of 8 participants that completed the study. The participants signed an informed consent document to participate in research involving human subjects according to the Declaration of Helsinki (WMA, 2014). The research project was also approved by the Ethics Committee in Research Involving Human Beings of the Galeão Air Force Hospital, Rio de Janeiro, Brazil, under protocol number (77577617.6.0000.5250).
Triathlon race's data collection
The data collection was realized during the Triathlon Endurance 2017 race of UFF Rio Triathlon circuit, in Recreio dos Bandeirantes, Rio de Janeiro City, Brazil. Table 1 shows the Triathlon race's structure and the measurements carried out.
Table 1 The Triathlon race's structure and the measurements carried out
Moment | Measurements |
---|---|
Baseline | Blood markers: creatine kinase (CK), lactate dehydrogenase (LDH), lactate, oxidative stress (Lipid peroxidation (LP), carbonylated proteins (CP), α-diphenyl-β-picrylhydrazyl (DPPH), total thiols (TT)), dehydration level, RPE Borg (0-10), Heat comfort, Skin wetness, Heat perception, Dry bulb globe temperature (C°), Wet bulb globe temperature (C°), Ocean temperature (C°), Relative Humidity (%) |
Swimming 1,5km | - |
1ᵃ Transition | RPE Borg (0-10), Heat comfort, Skin wetness, Heat perception, Dry bulb globe temperature (C°), Wet bulb globe temperature (C°), Relative Humidity (%) |
Cycling 60km | - |
2ᵃ Transition | RPE Borg (0-10), Heat comfort, Skin wetness, Heat perception, Dry bulb globe temperature (C°), Wet bulb globe temperature (C°), Relative Humidity (%) |
Running 15km | - |
Post-race | Blood markers: creatine kinase (CK), lactate dehydrogenase (LDH), lactate, oxidative stress (Lipid peroxidation (LP), carbonylated proteins (CP), α-diphenyl-β-picrylhydrazyl (DPPH), total thiols (TT)), dehydration level, RPE Borg (0-10), Heat comfort, Skin wetness, Heat perception, Dry bulb globe temperature (C°), Wet bulb globe temperature (C°), Relative Humidity (%) |
The baseline data collection occurred from 5:30 a.m. to 6 a.m. The triathlon race started at 6:50 a.m., and the post-race data collection occurred between 10 and 11 a.m., according to the total race time of each athlete. The minimum and maximum duration of the first transition was 3 and 5 minutes, and in the second transitions was 1 and 3 minutes, respectively.
Experimental procedures
Body composition and estimate maximum oxygen consumption (VO2max.) were measured in the Physical Education College of Brazilian Army (EsEFEx) laboratories.
Body mass was measured using a 150kg capacity scale with 100 g accuracy. Height was measured using a Filizola® (Brazil) stadiometer. It was used the procedures recommended by the International Society for the Advancement of Kinanthropometry - ISAK. Body Mass Index (BMI) was calculated by the ratio of body weight and the square of the height (kg/m2). The Jackson and Pollock protocol was used to assess body fat percentual (Jackson & Pollock, 1985).
The VO2max. was estimated by treadmill ergometric ramp protocol by ACSM (ACSM, 2018). Three minutes warm up to 9.0 km/h. Specific part increasing 0.5 km/h to every 30 seconds and 3 minutes cool down in 40% of maximum speed following American Heart Association recommendations (ACSM, 2014). The variables measured during the test were heart rate (HR) using HRM-Run™, HRM- Tri™, or HRM-Swim™ cardiac monitor, connect by pulse GPS Forerunner 920XT; Borg ratings of perceived exertion (RPE Borg (Borg, 2000). The temperature (T) and relative humidity (RH) were controlled in the lab (T=20,6°; RH=62%) following the recommendation of ACSM (ACSM, 2018).
One week before the triathlon race, these variables were collected to characterize the triathlon athletes’ fitness level, which participated in the present study.
Physiological markers
Blood markers of creatine kinase (CK), lactate dehydrogenase (LDH), lactate, oxidative stress (Lipid peroxidation (LP), carbonylated proteins (CP), α-diphenyl-ß-picrylhydrazyl (DPPH), total thiols (TT)), and dehydration level were collected in two moments: baseline and post-race. These two moments of data collection were determined not to affect the athletes’ performance and race time. Blood samples were collected using mineral-free needles (25 X 0,7 mm), the blood samples (14 ml) of the individuals were collected by the cubital vein, with the individuals remaining in a seated position in a tent. Mineral-free vacuum tubes and gloves were used, and the samples were transported to the laboratory for atomic absorption analysis and chemiluminescence immunoenzymatically assay to measure the serum levels concentrations(Marshall, Lapsley, Day, & Ayling, 2016). The automated biochemical Analyzer of the BT 3000 brand, manufactured by the company Wiener Lab. The recommendations of the commercial Kit (Wierner Lab.). The analysis procedures were performed in duplicate to confirm the results obtained.
After completion of all blood sample analysis procedures, the leftovers of laboratory samples containing blood were discarded in accordance with ANVISA legislation - RDC 306 of December 7, 2004, which provides on the technical regulation for waste management of health services.
Urinary and dehydration level. The urine collection of each volunteer was collected pre and post-race to compare to urine color index and analyzed the temperature and gravity of urine by the digital refractometer model RTD-95 (Instrutherm Ltda) measured (Minton, O’Neal, & Torres- McGehee, 2015). These two moments of data collection were determined not to affect the athletes’ performance and race time. The amount and type of liquid ingested by each athlete during the competition were free but controlled and measured at the end. Thermal stress control was registered using model TGD 400 (Instrutherm). The body mass before and using only underwear and all the athlete's clothing and accessories was also measured. For this measurement, it was used the balance Filizola ® Model PL 2007. The following equations were used:
Subjective perception variables
The thermal perception was measured by heat (score 1 to 7), comfort (score 1 to 6) and humidity (score 1 to 7) perception (Ackerley, Olausson, Wessberg, & McGlone, 2012), and the CR10 Borg Rate Perceived Exertion (RPE Borg), with a score between zero and 10 (Borg, 2000) was measure before the race, during transitions moments and post-race (Table 2). The researcher showed the printed visual analog scales for assessing thermal perception in different environments (Leon, Koscheyev, & Stone, 2008) at the moments described above. For the overall reliability, Cronbach's alpha value was 0.80, suggesting a normal distribution of the data and satisfactory internal consistency level (Taber, 2018).
Table 2 Exertion, heat, and environmental subjective perception scales of the participants.
Variables (scale) | Baseline | 1ᵃ Transition | 2ᵃTransition | Post-Race |
---|---|---|---|---|
RPE Borg (0-10) | 0.13±0.35 | 6.63±1.77* | 6.75±1.16* | 7.63±2.07* |
Heat comfort (1-6) | 2.38±0.74 | 2.25±0.46 | 3.00±1.41 | 4.13±1.25* # |
Skin wetness (1-7) | 1.13±0.35 | 5.75±1.16* | 3.88±0.99 | 4.63±0.74* |
Heat perception (1-7) | 2.50±0.53 | 3.63±1.06 | 5.00±1.31 | 6.00±0.53* |
*p<0.05 to Baseline;
#p<0.05 to 1ᵃ Transition.
Statistics
The statistical procedures were processed with the Statistical Package for the Social Sciences software (IBM SPSS Statistics 23, Chicago, USA). Descriptive statistics were applied to determine the mean, standard deviation, minimum and maximum values. The Shapiro-Wilk (SW) test was used to verify the normality of data. Paired Student's t-test was used to analyze the intragroup comparisons to physiological and biochemical variables on baseline and post-race. Freedman's ANOVA followed by Post Hoc of Dunn was used to compare the scores of CR10 Borg, Heat comfort, Skin wetness, and Heat perception on different moments (Baseline, 1a transition, 2a transition, and Post-race). The effect size of Cohen (d) was measured to analyze the magnitude of a treatment effect. It was classified into small (d ≤ 0.2); medium (0.2 < d < 0.79) and large (d ≥ 0.8) (Cohen, 2013). The significance level was p<0.05.
RESULTS
The mean age of the triathlon athletes was 32.88±4.31years old (SW p=0.429), presented a total body mass of 78.46±8,10 kg (SW p=0.972), body fatness of 6.70±0.93 % (SW p=0.163), and maximum oxygen consumption of 69.61±3.81 mlO2/ kg/min (SW p=0.223). During the triathlon race, the ocean temperature remained at 18 ° C and the relative humidity at 60%. There was a variation between the ambient temperature (15.9 - 22.6 ° C) and the heat stress (15.9 - 23.2 ° C). No change in the relative humidity was observed. It could be observed in Table 2 the heat, environmental, and exertion perception and data collect during the Triathlon race.
It was observed a significant increase in RPE Borg on 1ᵃ transition (p< 0.001; d= 18.6), 2° transitions (p< 0.001; d= 18.9) and post-race (p< 0.001; d= 21.4). There was a significant increase in baseline heat comfort to post-race (p= 0.013; d= 2.4) and in the 1ᵃ transition to post-race (p= 0.007; d= 4.1). Skin wetness baseline presented a significant increase to 1a transition (p< 0.001; d= 13.2) and to post-race (p< 0.001; d= 10.0). And the baseline Heat perception showed a significant increase to post-race (p< 0.001; d= 6.6).
The Figures 1 presents blood markers of CK, LDH and lactate of sample. The variables of injury and muscle fatigue CK (p= 0.005; d= 5.7), LDH (p= 0.004; d= 1.3) and lactate (p< 0.001; d= 7.2) presented a significant increase comparing the baseline and post-race data.
The Figures 2 presents blood markers of oxidative stress (Lipid peroxidation (LP), carbonylated proteins (CP), α-di-phenyl-ß-picrylhydrazyl (DPPH), and total thiols (TT) of sample. It was observed a significant increase in CP (p= 0.021; d= 2.2) and a significant decrease in DPPH (p= 0.002; d= −1.4) in post-race. No significant changes in LP (p= 0.217; d= 0.6) and TT (p= 0.881; d= −0.1) oxidative stress markers variables was verified.
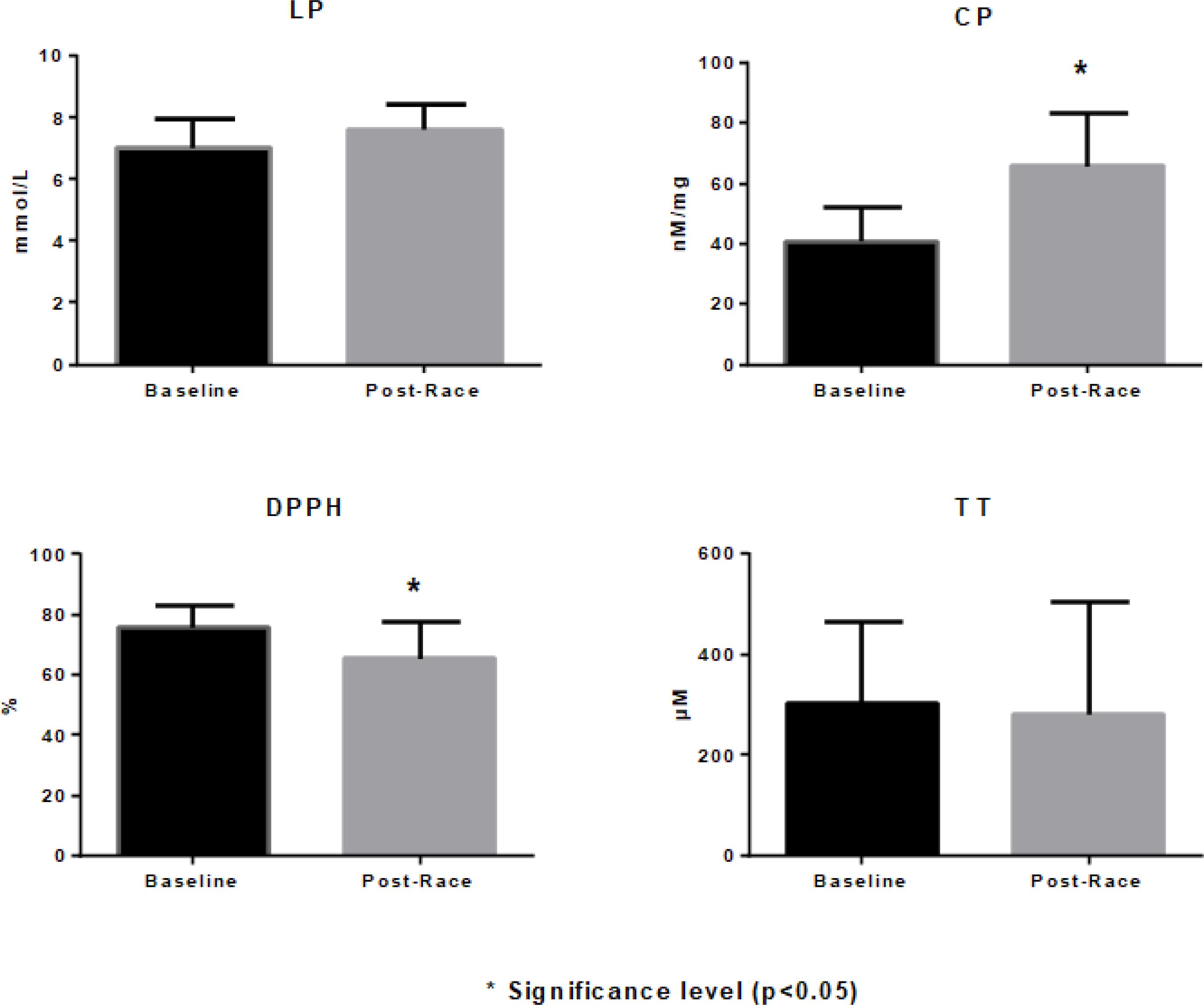
Figure 2 Blood markers of oxidative stress (Lipid peroxidation (LP), carbonylated proteins (CP), α-diphenyl-β-picrylhydrazyl (DPPH), and total thiols (TT) of the participants.
Table 3 presents the dehydration levels comparing baseline and post-race. A significant increase (Δ% = 30.95, p = 0.001; d = 6.8) was observed in urine temperature and color (range [1-7], p = 0.039). However, urine gravidity did not change (Δ% = 0.00, p = 1.000). The mean race time was 3.64 ± 0.407 hours, which was within the maximum time for a race with its characteristics, 5.30 hours. The mean sweating rate was 1925.0 ± 919.27 mL, and the estimated sweating rate (mL/h) was 545.0 ± 0.293, which represents approximately 2 kg of weight loss during a test. No significant correlation (p <0.05) was observed between the Environmental Perception Scales and Ambient Temperature and the athlete's performance.
Table 3 Dehydration level of the participants
Variables | Baseline (Mean ± SD) | Post-race (Mean ± SD) | Δ% (p value) |
---|---|---|---|
Urine temperature | 18.08 ± 0.84 | 23.75 ± 2.06* | 30.95 (p=0.001) |
Urine gravidity | 1.337 ± 0.004 | 1.337 ± 0.002 | 0.00 (p=1.000) |
Urine color (mode) | 1 | 7* | 85.70 (p=0.039) |
Hematocrite (%) | 45.61 | 45.77 | 0.36 (p=0.801) |
*significance level (p<0.05)
DISCUSSION
The participants presented an excellent body composition and aerobic fitness within their respective reference (ACSM, 2014). This fact may have contributed to the accomplishment of the race within the deadline stipulated by the organization of the event (5.30 hours), where the mean race time was 3.64 ± 0.41 hours (Dengel, Flynn, Costill, & Kirwan, 1989; O’Toole & Douglas, 1995).
It was observed the increase of effort perception and heat perception with the reduction of heat comfort and skin wetness in the present study. The same tendency to increase the perceived effort as the triathlon race progresses occurred in a study with twelve triathlon athletes in an Ironman race (Puggina, 2008). The association between the increase of effort perception with the reduction of heat comfort is explained due to the increase of the body temperature as a result of the physical activity (McArdle, Katch, & Katch, 2016). The increase in the heat perception during the race, even with the ambient temperature beneath the values considered for heat stress (23.7°C), is related to the stress of the exercise and thermoregulation (Ackerley, et al., 2012).
The greatest risk for heatstroke occurs during prolonged high-intensity exercise when the wet-bulb temperature exceeds 28°C (ACSM, 2014). According to the heat index related to heat sensation, alert descriptions, and recommended sports activities, warm temperatures between 18-24°C require precautions, hot temperatures between 24-28°C require extreme caution, and very hot temperatures above 28°C are dangerous (Brocherie & Millet, 2015).
In our study, the ambient temperature (15.9 - 22.6 °C) and the heat stress (15.9 - 23.2 °C) were below it, so the athletes were exposed to no thermal stress risks. Endurance performance during whole-body exercise is known to be impaired in the heat, and that subjective thermal strain is an important and independent mediator of the heat-induced impairment in endurance performance (Van Cutsem, Roelands, De Pauw, Meeusen, & Marcora, 2019). Triathletes with hyperthermia during the marathon of Ironman World Championship in Kona run more slowly (Olcina, Crespo, Timón, Mjaanes, & Calleja-González, 2019).
Regarding the blood markers, the CK, LDH, and lactate presented a significant increase comparing the baseline and post-race data. A similar study with 23 athletes who completed the Ironman in Johannesburg (1983) presented a significant increase in CK activity post-race and changes in other biochemical indicators such as glucose, fatty free acids, and lactate (Van Rensburg, Kielblock, & Van der Linde, 1986). It was also observed in a 70.3 Ironman race, an increase of CK and LDH post-race in 12 volunteer athletes (Puggina, 2008). Another study performed with 12 athletes, similar to Ironman, presented a high activity of LDH postrace (Margaritis, Tessier, Verdera, Bermon, & Marconnet, 1999). It was noticed the oxidative damage, inflammation, and increase of CK and LDH (Clifford et al., 2016) even in different race distances.
Olcina et al. (2018) reported a significant change in blood variables after the Olympic triathlon race as the muscle damage caused by an increase in blood levels of LDH and CPK. However, the increases observed were lower than in others races due to the distance and duration of the olympic race. These data suggest that muscle damage occurs after the Olympic distance triathlon but less than in triathlons of longer distances (Olcina, et al., 2018). In our study, the distances of endurance triathlon race were higher (1.5 km swimming, 60km cycling, 15 km running).
The tissue damage most common causes are neutrophil infiltration, calcium ion imbalance, free radical production, and excretion of cytokines (Withee et al., 2017), and the antioxidant defenses of the body are usually adequate to prevent substantial tissue damage (Cooper, Vollaard, Choueiri, & Wilson, 2002). Other factors are also associated with physical exercise, such as tissue inflammation. This factor can lead to oxidative stress and contribute to performance loss, fatigue, and muscle injury(Petersen & Pedersen, 2005). Moreover, the high-volume and high-intensity training combined with the emotional and physiologic stress caused by the competition could influence the immunological and hormone responses (Olcina, et al., 2019).
Usually, the antioxidant system is not enough to eliminate oxidative damage during and after exercise, although the body's complex antioxidant defense system (Pinho et al., 2010). Oxidative damage in exercise depends on its type and intensity. However, studies have shown that endurance training improves antioxidant defense as well as oxidative capacity in skeletal muscle (Pereira, Rosa, Safi, Bechara, & Curi, 1994). The markers of oxidative stress in our study suggested a good antioxidant defense.
Cutter at al. (2019) evaluated the effects of strenuous exercise and the risk of oxidative stress in eleven well-trained triathletes scheduled to compete in the Full Ironman Placid or Ironman Placid Half for summer 2017 and summer 2018. The athletes who completed the full Ironman triathlon experienced increased amounts of oxidative stress denoted by the elevated cortisol levels, increased 8-oxo-dG concentrations, and increased ROS concentrations (Cutter, Cruz, Cristall III, Lacey, & Julian, 2019). In the present study, the significant increase in CP and decrease in DPPH post-race and no significant changes in oxidative stress markers LP and TT seems to contribute to the idea of improvement of antioxidant defense as triathlon training adaption.
A systematic review and meta-analysis that aimed to identify biomarkers associated with altered exercise performance following intensified training concluded that many biomarkers were altered with intensified training but not in a manner related to changes in exercise performance(Brocherie & Millet, 2015).
Kim et al. (2018) reported that the high-intensity long-term aerobic exercise triathlon leads to temporary weight loss, DNA damage, and muscle damage after the race and such changes are affected by exercise duration and intensity. Furthermore, during these changes, the defense mechanisms to protect against DNA and muscle damage, such as anti-oxidant defenses, may also occur during recovery and race (Kim et al., 2018). Seems to exist a dose-response relationship between adaptations of antioxidant enzymes and responses to ultra-endurance exercise, related to volumes and intensities of activity associated with ultra-endurance training confer protection against increases in free radical damage, resulting in improved oxidative balance (Ackerley, et al., 2012). We could also observe in our study the improvement of endurance training in the antioxidant defense.
Other relevant data, the total body mass of the triathletes in our study reduced by approximately 2 kg, characterizing dehydration. The effects of dehydration can occur even with a moderate reduction in total body mass (1-3%)(Armstrong, Costill, & Fink, 1985). Dehydration between 1.9 and 4.3% of total body mass can reduce resistance by 22-48% and VO2max by 10-22% (Whyte, et al., 2015). Moreover, there is an increased risk of heat illness if the exercise is performed in a state of dehydration (Mello, 2018).
Although the urine density values did not change, they were above the pre-test reference values, however, these values did not suffer a significant change in the post-test. While a significant increase in color was observed post-race, characterizing as “very dehydrated”, even with an orientation of free hydration during the race. It is an important alert that can compromise the performance. James et al. (2019) stated that hypohydration seems to impair endurance performance through a combination of mechanisms, driven by hypovolemia and these physiological and perceptual responses due to the increased perception of effort compromising the performance (James, Funnell, James, & Mears, 2019). So, athletes’ health and performance are dependent on avoidance of performance, limiting hypohydration or overhydration (Hoffman, 2019).
It can be highlighted that the triathlon is a single modality and not as the sum of three distinct sports modalities, and the present study demonstrated the impact of an endurance triathlon race on physiological markers and subjective perception variables in amateur triathletes. The limitations are the small number of participants because most of the selected athletes were not volunteers, assuming that the study protocol could influence their race performance. It was not controled the daily activities, the total amount of training, and sleeping hours during the week race. Furthermore, for future research, it is suggested a larger sample and evaluate other types of triathlon race, like Ironman.
CONCLUSIONS
It can be concluded that the acute effects of the endurance triathlon race on variables of injury and muscle fatigue presented an increase comparing the baseline and post-race data. The same behavior was observed in carbonylated proteins (CP), α-diphenyl-ß-picrylhydrazyl (DPPH), and a decrease in α-diphenyl-ß-picrylhydrazyl (DPPH).
Regarding the subjective perception variables, a significant increase in RPE Borg was observed along the moments of the race. There was an increase in heat comfort and heat perception in post-race compared with baseline.
The knowledge produced in this study can be applied in a practical way to control training intensity (training load control) and as a reference of these biomarkers for the modality. It can be recommended: hydration mainly after swimming and during cycling due to the masking of the thermal sensation at these times; active recovery with the bicycle on the roller or light running is recommended for better post-race enzymatic recovery; and application of recovery scales and late muscle pain, as well as the reduction of the total training volume, when the biochemical markers of injury and tissue inflammation are elevated after the competition or training.