Serviços Personalizados
Journal
Artigo
Indicadores
-
Citado por SciELO
-
Acessos
Links relacionados
-
Similares em SciELO
Compartilhar
Revista de Ciências Agrárias
versão impressa ISSN 0871-018X
Rev. de Ciências Agrárias vol.42 no.1 Lisboa mar. 2019
https://doi.org/10.19084/RCA18140
ARTIGO
Physiological response of Physalis peruviana L. seedlings inoculated with Funneliformis mosseae under drought stress
Respostas fisiológicas de mudas de Physalis peruviana L. inoculadas com Funneliformis mosseae sob déficit hídrico
Sergio Manuel Rugeles Reyes1,*, Gabriel Roveda Hoyos2, Domingos da Costa Ferreira Júnior1, Arthur Bernardes Cecílio Filho1 and Liz Patricia Moreno Fonseca2
1 Universidade Estadual Paulista Julio de Mesquita Filho. Jaboticabal (SP), Brasil
2 Universidade Nacional de Colômbia. Bogotá, Colômbia
(* E-mail: smrugelesr@gmail.com)
ABSTRACT
Physalis peruviana L. is gaining importance in international markets due to its nutritional properties; however, the production regions are increasingly susceptible to drought periods due to global climate change. A sustainable tool against this abiotic constraint is the association with arbuscular mycorrhizal fungi (AMF). The aim of this work was to evaluate the effects of AMF inoculation with Funneliformis mosseae on the early development and some physiological parameters of P. peruviana under drought stress. The tested treatments were three soil water conditions (30%, 40% and 80% of field capacity) and the presence or absence of AMF. In this sense, root colonization quantification, root and shoot dry weight, relative water content (RWC), leaf water potential, net photosynthesis (A), transpiration (E), stomatal conductance (gs), electrolyte leakage, chlorophyll content and fluorescence were evaluated. The treatments representing 30% and 40% of field capacity had a negative effect on all the evaluated parameters; nevertheless, AMF plants presented enhanced values of root dry weight, RWC, A, gs and chlorophyll content in comparison to non-inoculated stressed plants. Despite this, mycorrhization did not affect stress indicators as chlorophyll fluorescence and electrolyte leakage, revealing that, although AMF is a promising strategy, it is not enough to relieve drought stress deleterious effects, and need to be integrated with other management strategies.
Keywords: arbuscular mycorrhizal, cape gooseberry, climate change, gas exchange, water deficit.
RESUMO
Physalis peruviana L. vem adquirindo importância nos mercados internacionais devido a suas propriedades nutricionais, entretanto diante das mudanças climáticas suas regiões de cultivo são cada vez mais suscetíveis a períodos de seca. Uma estratégia para essa restrição climática é a associação com fungos formadores de micorrizas arbusculares (MA). O objetivo deste estudo foi avaliar os efeitos da inoculação com o fungo Funneliformis mosseae sobre o crescimento inicial e alguns parâmetros fisiológicos de P. peruviana L. sob déficit hídrico. Os tratamentos testados foram três regimes hídricos (30%, 40% e 80% da capacidade de campo) e presença ou ausência de MA. Nesse sentido, a quantificação da colonização, massa seca da raiz e da parte aérea, conteúdo relativo de água (CRA), potencial hídrico foliar, taxa de fotossíntese (A), transpiração (E), condutância estomática (gs), conteúdo e fluorescência de clorofilas e extravasamento de eletrólitos foram avaliados. Determinou-se efeito negativo dos tratamentos com 30% e 40% de capacidade de campo para todas as variáveis, porém, plantas com AM apresentaram maiores valores de massa seca de raiz, CRA, A, gs e conteúdo de clorofilas. Contudo, não houve efeito da micorrização na fluorescência da clorofila e extravasamento de eletrólitos, indicando que apesar de a simbiose ser promissora, não é suficiente para atenuar os efeitos deletérios do déficit hídrico como estratégia isolada, e deve ser integrada com outras estratégias de manejo.
Palavras-chave: micorrizas arbusculares, physalis, mudança climática, trocas gasosas, déficit hídrico.
INTRODUCTION
Physalis peruviana L., popularly known as Cape gooseberry or physalis, is a species originated from the Andes region, belonging to Solanaceae family. This plant is grown as an exotic fruit in Ecuador, Kenya, Zimbabwe, Malaysia, Brazil and Colombia, the latter being the largest producer in the world (Agronet, 2016). The fruit of P. peruviana has high contents of vitamin C, proteins and vitamin A (Ramadan, 2011) and bioactive compounds, such as withanolides, glycosides and physalins, being used in traditional medicine for cancer and leukemia treatments (Yen et al., 2010). Due to these properties, physalis has presented a large potential for the international markets.
In Colombia, commercial production comprehends an area of approximately 1000 ha (Analdex, 2017) and is concentrated in mountain regions of Cundinamarca, Boyacá and Antioquia, which represents 95% of national production (Agronet, 2016). In this country, P. peruviana is the second most exported fruit after bananas; however, in the last years exports have decreased in 13.6%, and this can be related to environmental conditions (Analdex, 2017). In the main physalis producing regions of Colombia, temperature has increased by about 0.5°C and rainfall has decreased by 60% in the last 50 years (Pabon, 2012). This situation may get even more concerning, as is expected for Cundinamarca and Boyaca regions drastic rainfall reductions until 2050, with higher occurrence of drought periods (PRICC, 2014). Therefore, physalis production in Colombia has upcoming challenges, since the national production is mostly in fields that rely on rainfall to meet crops hydric demand (Fisher & Miranda, 2012).
Drought is considered the most restrictive abiotic factor to crop growth, development and yield (Zhou et al., 2015), as it impairs several metabolic parameters, such as electron transport chain, reactive oxygen species (ROS) homeostasis (Mirshad & Puthur, 2017), photosynthesis and carbon metabolism (Yooyongwech et al., 2016), tissue water and chlorophyll contents (Abbaspour et al., 2012). However, plants have developed a wide range strategies to overcome this stress, as symbiotic association with arbuscular mycorrhizal fungi (AMF), which is frequently reported augmenting drought tolerance in different species (Zhou et al., 2015; Yooyongwech et al., 2016; Amiri et al., 2017).
AMF are recurrent in 90% of the vascular plant species (Brundrett, 2009). In mycorrhizal associations, plants provide the fungus carbohydrates from photosynthesis, receiving in return higher uptake of water and nutrients like phosphorus, calcium, sulfur, zinc and iron due to fungal hyphae absorption (Kara et al., 2015). Among the physiological benefits to plants from mycorrhizal association, there are reports of higher stomatal conductance, phosphorus absorption, photosynthesis rate and antioxidant enzyme activity (Auge et al., 2015; Mirshad & Puthur, 2017). There are also positive reports for P. peruviana L., as increased growth under salt stress, improved photosynthetic efficiency and dry weight accumulation (Miranda et al., 2011). Nonetheless, there is still little information about the relationship between drought stress and mycorrhizal association for this species at seedling stage, as most studies have been focusing on the stress effects on fruit quality (Gordillo et al., 2004; Alvarez et al., 2012).
In face of the increasing importance of this crop in the international market and the growing risk of water shortage in production areas, the aim of this study was to assess physiological responses of Physalis peruviana L. inoculated with the AMF Funneliformis mosseae under different levels of water deprivation.
MATERIAL AND METHODS
Installation and growth conditions: the experiment was carried out between November 2013 and February 2014 in greenhouse conditions at National University of Colombia, Bogotá campus (4°3556 N, 74°0451 W and altitude of 2556 m). P. peruviana L. seedlings (cv. Colombia) were obtained in germination chambers with average relative humidity of 80%, daytime and nighttime temperatures of 28oC and 18oC, respectively, and 12-hour photoperiod. Twenty days after sowing, seedlings with three leaves were transplanted for 0.5 L pots filled with substrate composed by equal parts of granite, river sand and coarse sand.
Fertigation began one week after seedling transplant with Hoagland & Arnon (1950) nutrient solution modified to 80% of its original concentration (N=179,5 mg L-1; P=50 mg L-1; K=188,16 mg L-1; Ca= 128 mg L-1; Mg= 19,2 mg L-1; Cl= 1,4mg L-1; B=0,21 mg L-1; Mn=0,08 mg L-1; Zn=0,104 mg L-1; Cu=0,03 mg L-1; Mo=0,05 mg L-1 and Fe= 1,5 mg L-1). Substrate moisture was kept at 80% of its field capacity for 60 days after transplant (DAT), when the drought treatments were started.
Arbuscular mycorrhizal fungi (AMF) inoculum production: the chosen species was Funneliformis mosseae (previously known as Glomus mosseae), Gerd. & Trappe (BEG 12), based on preliminary trials to determine the best species for P. peruviana. Plants were inoculated at two moments: at seedling transplant and one week after. To obtain F. mossae inoculum, the fungus was reproduced in pots filled with coconut fiber, using Brachiaria decumbens as host species. Plants were harvested one month after germination, discarding aboveground part. AMF consisted in soil, spores (50 spores g-1 soil, approximately) and fragments of infected roots.
Experimental design and treatments: the assessed factors were soil moisture levels (30%, 40% and 80% of field capacity [FC], considering the latter as control) and mycorrhization (with and without inoculation). To control soil moisture levels, five check pots with growing P. peruviana plants were watered until field capacity and weighed, and in the following day, the water loss by evapotranspiration was calculated. Then, 30%, 40% and 80% of this amount was daily supplied to pots. It was adopted a randomized blocks design and a 3x2 factorial layout, with four replicates. The experimental unity consisted in five plants per replicate.
Assessed parameters
Quantification of root colonization: once concluded the experiment, roots from all plants were taken for the determination of AMF colonization, according to methodology proposed by Giovanetti & Mosse (1980).
Biomass: to assess root and shoot dry weight, collected plants were separated with a scissor into roots and shoots, then set in paper bags to dry in an oven with forced air circulation at 65oC, until the achievement of constant weight. Then, each sample was weighed in a precision balance.
Hydric status: relative water content (RWC) was determined on the 4th leaf (from the apex), as described by Schonfeld et al. (1988) and leaf water potential (Ψh) was measured on the 5th leaf, using a Scholander pressure chamber (model 1000 PMS Instrument Company).
Gas exchange: to evaluate net photosynthesis rate (A), transpiration rate (E) and stomatal conductance (gs), it was used the equipment LCpro-SD Portable (Photosynthesis System-BioScientific), with a radiation level of 900 μmol m-2 s-1. All the readings were performed on the 5th leaf (from the apex), between 8:00 and 11:00 a.m.
Chlorophyll content: chlorophyll content was determined through a SPAD meter (Chlorophyll Content Meter, model CC-200 plus) on the 4th leaf (from the apex).
Chlorophyll fluorescence: this parameter was determined with a MiniPAM portable pulse modulated fluorometer (Walz, Effeltrich, Germany). Readings were performed in leaves previously acclimatized to darkness for 3 hours, applying pulses of 1900 μmol photon m-2 s-1 to completely saturate photosystem II (PSII) reaction center. PSII activity was obtained through measurements performed at 8:00 p.m. of the maximum quantum yield of PSII.
Membrane stability: it was assessed through the evaluation of electrolyte extravasation (EL). Ten 1-cm diameter discs from the 5th leaf were placed in test tubes containing deionized water. After eight hours, electric conductivity of the solution was measured with a conductivity meter. Twenty-four hours later, tubes were heated at 80oC for 15 minutes and electric conductivity was measured once more to determining electrolyte leakage according to Fan & Blake (1994).
The evaluation of root colonization quantification and dry weight were performed 120 DAT, when 80% of the plants reached 20 cm height, which is the recommended moment for definitive transplant into the field (Oliveira et al., 2017). The other variables were analyzed at 105 and 120 DAT.
Statistical analysis: Data were analyzed by two-way analysis of variance (ANOVA), followed by Tukeys HSD (p≤0.05) for mean values comparison, through the software SAS version 9.1.
RESULTS AND DISCUSSION
All plants inoculated with F. mosseae (AMF) presented root colonization, whilst non-inoculated replicates did not present association with the fungi (Table 1). Colonization rates were higher in plants under 80% field capacity (FC) in comparison to 30% and 40%, which presented values 60% and 46% lower, respectively. Similar results were found by Abbaspour et al. (2012) and Amiri et al. (2017) for Pistacia vera L. and Pelargonium graveolens, respectively. The decreased root colonization by the fungus can be explained by lower spore germination and hyphae growth due to water limitation (Gong et al., 2013).
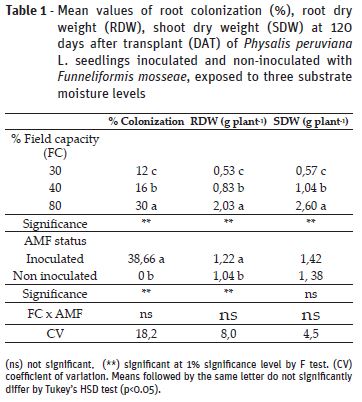
Root dry weight (RDW) was significantly affected by FC treatments and inoculation; however, interaction between these factors was not significant. Plants under 30% and 40% FC had an defective root development, represented by RDW values 73% and 59.1% lower than the ones grown under 80% FC, suggesting that root weight accumulation depends on the severity of the drought stress. On the other hand, AMF inoculated plants had an enhancement of 17% in comparison to non-inoculated counterparts (Table 1). For shoot dry weight (SDW) there was significant effect of the different soil moisture levels, but not from the inoculation (Table 1). The impairment of dry weight accumulation due to drought stress is a result of decreased leaf water potential, cell division and nutrient absorption (Zhao et al., 2015). In this work, it was noticed that AMF induced higher RDW accumulation, what may allow plants to explore hydric resources in deeper layers of the soil and tolerate periods of water scarcity (Lynch, 2013). Therefore, enhanced root dry weight accumulation observed in the present work might contribute to better shoot growth in further developmental stages. Despite that, at the evaluated moment it was not observed significant effect of the symbiosis on shoot dry weight, as also reported by Miranda et al. (2011), who observed AMF effect on developmental stages near from flowering and fruitification, but not at vegetative growth.
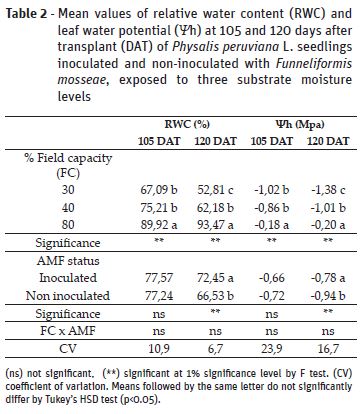
Relative water content (RWC) and leaf water potential (Ψh) values were reduced as a consequence of the soil moisture levels at the two assessed moments (Table 2). There were no significant effects of inoculation on RWC at 105 days after transplant (DAT); however, at 120 DAT it was observed an increase of 9% in plants with AMF, in comparison to non-inoculated counterparts. Observing Ψh results, there was significant effect of inoculation on plants at 120 DAT, which presented higher value than non-inoculated plants. Lower RWC and Ψh in plants under 30% and 40% FC are results of lower cell turgor and stomatal closure to prevent excessive water loss under drought stress (Kramer & Boyer, 1995). The improved RWC and Ψh values by mycorrhiza at 120 DAT indicates that inoculation favored water movement from roots to leaves, as observed for other species through improved root hydraulic conductivity by fungi hyphae and higher water and nutrients uptake (Ortiz et al., 2015, Yooyongwech et al., 2016)..
Table 3 presents net photosynthesis (A), transpiration (E) and stomatal conductance (gs) rates. At 105 DAT, net photosynthesis rate was 52% and 32% lower under 30% and 40% FC, respectively, in comparison to plants under 80% FC, without significant effect of F. mosseae inoculation. However, at 120 DAT there was significant effect from inoculation, resulting in an improvement of 25.6% of A in AMF-associated plants compared to the non-inoculated replicates. Similar responses were detected for E and gs, for which were observed effect of drought stress treatments at 105 e 120 DAT, while inoculation effect was significant only at 120 DAT..
The restraint of gas exchange parameters under drought stress is a negative consequence of stomatal closure to reduce water loss, which also results in lower carbon use efficiency and oxidative damage by light (Erice et al., 2011). However, our results demonstrate that F. mossae inoculation alleviates these deleterious effects at 120 DAT, suggesting that mycorrhization benefits water relations in plants, enabling the maintenance of stomatal aperture and proper plant transpiration (Auge et al., 2015), as also observed in other species (Gong et al., 2013; Amiri et al., 2017). Quiroga et al. (2017) proposed that AMF genetically modifies root hydraulic properties, improving stomatal conductance, CO2 diffusion to carboxylation sites and photosynthetic rate, which were observed in the present study. Higher photosynthetic rate could also be a result of increased phosphorus absorption by inoculated plants (Amiri et al., 2017). Additionally, it is highlighted that the obtained results are in accordance to the ones found for hydric status (RWC and Ψh Table 2), demonstrating the close association between these parameters.
In this experiment, it was observed lower chlorophyll contents at 105 DAT in plants under 30% and 40% FC compared to 80% FC (Table 4). This fact was clearer at 120 DAT, when plants under 30% and 40% FC presented chlorophyll contents respectively 27.2% and 17.45% lower than non-stressed plants. Similar results were reported by Deveci & Celik (2016), who found for the same species 26.6% and 32.9% of SPAD values for plants irrigated until 25% and 50% of FC, respectively. Stomatal closure induced by drought, as observed in this work (Table 3), promotes lower CO2 influx, modifying electron transport chain and enhancing NADPH reduction potential, leading to a rise in superoxide radicals production and inducing oxidative damage in chlorophyll molecules (Mittler, 2017).
Despite not being significant at 105 DAT, AMF resulted in 8% higher chlorophyll contents at 120 DAT compared to non-inoculated plants. These results indicate that plants associated with AMF have lower chlorophyll degradation under drought stress or higher synthesis of this pigment as a result of enhanced nutrient absorption (Abbaspour et al., 2012; Amiri et al., 2017). The obtained chlorophyll results could also be a reason for the ameliorative effect of AMF on A at 120 DAT (Table 3).
Despite the ameliorative effects of AMF observed on RDW and photosynthetic parameters, the evaluated stress indicators did not show significant effects from the fungi association (Table 4). PSII quantum yield, also denoted as Fv/Fm, is used to assess its primary photochemical capacity and a strong indicator of biotic and abiotic disturbances (Qiu et al., 2012). At 105 DAT, 30% and 40% FC resulted in a decrease of approximately 20% in comparison to the highest substrate moisture. The decline of Fv/Fm as a drought stress consequence was even more evident at 120 DAT, when plants under 30% and 40% FC had 40% e 36% lower values, respectively, in comparison to plants under 80% FC. At both evaluation moments it was not observed significant effect of F. mosseae inoculation, just like reported by Zhou et al. (2015) for inoculated wheat seedlings.
Fv/Fm results under 80% FC demonstrate that plants in this condition presented normal functioning, as the observed values were superior to 0.75, which is defined as the reference value to indicate stress (Mirshad & Puthur, 2017). On the other hand, 30% and 40% FC induced damages to PS II, with Fv/Fm values close to 0,5. This decrease shows that drought stress undermines the structure and functioning of the PSII, reducing carbon assimilation due to its hyperexcitation and photoinhibition (Kitao & Lei, 2007), besides from impairing the electron transport chain (Mirshad & Puthur, 2017).
Electrolyte leakage (EL) reflects loss of cell membrane integrity, consequence of lipid peroxidation induced by drought stress (Fouad et al., 2014). In the present investigation, 30% FC resulted in the highest electrolyte leakage, which was even higher in following evaluation moments, independent from AMF (Table 4). This result is comparable to Deveci & Celik (2016), who found EL values of 69%, 47% and 38% under soils at 25%, 50% and 75% FC, respectively. Despite there was no significant effect of mycorrhization, studies with other species report that AMF can improve and restore cell membrane integrity under drought stress by different pathways (Fouad et al., 2014; Ortiz et al., 2015). However, AMF effects are very complex and varies with the environment, plant and fungi species (Zhou et al., 2015), and the present results suggest that in the case of P. peruviana, inoculation alone was not enough to ameliorate the oxidative stress induced by water deprivation, although its beneficial effects on some parameters that allow plant growth under drought stress..
CONCLUSION
The results found in the study showed that the inoculation with F. mosseae in plants of P. peruviana L. under drought stress promoted beneficial effects in the accumulation of root dry matter, in the hydric state of the plant and also, improves the parameters related to gas exchange, which leads to higher photosynthetic rate. Although AMF alone was not enough to attenuate the deleterious effects of oxidative stress due to water deficit, it is highlighted that the inoculation with F. mosseae combined with other management practices, such as the use of other beneficial microorganisms or mycorrhiza species, could become a successful management strategy for physalis producers in drier environments.
References
Abbaspour, H.; Saeidi-Sar, S.; Afshari, H. & Abdel-Wahhab, M.A. (2012) - Tolerance of mycorrhiza infected pistachio (Pistacia vera L.) seedling to drought stress under glasshouse conditions. Journal of Plant Physiology, vol. 169, n. 7, p. 704-709. https://doi.org/10.1016/j.jplph.2012.01.01. [ Links ]
Agronet (2016) - Red de información y comunicación del sector agropecuario Colombiano. [cit. 2018-08-18]. <http://www.agronet.gov.co/Paginas/default.aspx> [ Links ].
Alvarez, J.; Balaguera, H. & Fischer, G. (2012) - Effect of irrigation and nutrition with calcium on fruit cracking of the cape gooseberry (Physalis peruviana L.) in the three strata of the plant. Acta Horticulturae, vol. 928, p. 163-170. https://doi.org/10.17660/ActaHortic.2012.928.19 [ Links ]
Amiri, R.; Nikbakht, A.; Rahimmalek, M. & Hosseini, H. (2017) - Variation in the essential oil composition, antioxidant capacity, and physiological characteristics of Pelargonium graveolens L. inoculated with two species of mycorrhizal fungi under water deficit conditions. Journal of Plant Growth Regulation, vol. 36, n. 2, p. 502-515. https://doi.org/10.1007/s00344-016-9659-1 [ Links ]
Analdex (2017) - Comportamiento de la uchuva- Producción y comercio. Asociación Nacional de Comercio Exterior. [cit. 2018-08-18]. <http://www.analdex.org/wp-content/uploads/2018/02/2018-02-08-Mercado-de-la-Uchuva.pdf> [ Links ]
Auge, R.; Toler, H. & Saxton, A. (2015) - Arbuscular mycorrhizal symbiosis alters stomatal conductance of host plants more under drought than under amply watered conditions: a meta-analysis. Mycorrhiza, vol. 25, n. 1, p. 13-24. https://doi.org/10.1007/s00572-014-0585-4 [ Links ]
Brundrett, M. (2009) - Mycorrhizal associations and others means of nutrition of vascular plants: understanding the global diversity of host plants by resolving conflicting information and developing reliable means of diagnosis. Plant and Soil, vol. 320, n. 1-2, p. 37-77. https://doi.org/10.1007/s11104-008-9877-9 [ Links ]
Deveci, M. & Celik, A. (2016) - The effects of different water deficiency on physiological and chemical changes in Cape gooseberry (Physalis peruviana L.) which were grown in greenhouse conditions. Scientia Agriculturae, vol. 14, n. 2, p. 260-265. https://doi.org/10.15192/PSCP.SA.2016.14.2.260265 [ Links ]
Erice, G.; Louahlia, S.; Irigoyen, J.; Sanchez-Diaz, M.; Alami, T. & Avice, J. (2011) - Water use efficiency, transpiration and net CO2 exchange of four alfalfa genotypes submitted to progressive drought and subsequent recovery. Environmental and Experimental Botany, vol. 72, n. 2, p. 123-130. https://doi.org/10.1016/j.envexpbot.2011.02.013 [ Links ]
Fan, S. & Blake, T. (1994) - Abscisic acid induced electrolyte leakage in woody species with contrasting ecological requirements. Physiologia Plantarum, vol. 90, n. 2, p. 414-419. https://doi.org/10.1111/j.1399-3054.1994.tb00407. [ Links ].
Fischer, G. & Miranda, D. (2012) - Manual para el cultivo de frutales en el trópico. 1 ed. Produmedios, Bogotá. 1023 p. [ Links ]
Fouad, M.; Essahibi, A.; Benhiba, L. & Qaddoury, A. (2014) - Effectiveness of arbuscular mycorrhizal fungi in the protection of olive plants against oxidative stress induced by drought. Spanish Journal of Agricultural Research, vol. 12, n. 3, p. 763-771. http://dx.doi.org/10.5424/sjar/2014123-4815 [ Links ]
Giovannetti, M. & Mosse, B. (1980) - An evaluation of techniques for measuring vesicular-arbuscular infection in roots. New Phytologist, vol. 84, p. 489-500. https://doi.org/10.1111/j.1469-8137.1980.tb04556. [ Links ]
Gong, M.; Tang, M. & Chen, H. (2013) - Effects of two Glomus species on the growth and physiological performance of Sophora davidii seedlings under water stress. New Forests, vol. 44, n. 3, p. 399-408. https://doi.org/10.1007/s11056-012-9349-1 [ Links ]
Gordillo, O.P.; Fischer, G. & Guerrero, R. (2004) - Efecto del Riego y de la fertilización sobre la incidencia del rajado en frutos de uchuva (Physalis peruviana L.) en la zona de Silvania (Cundinamarca). Agronomía Colombiana, vol. 22, n. 1, p. 53-62. [ Links ]
Hoagland, D.R. & Arnon, D.I. (1950) - The water culture method for growing plants without soil. California Agricultural Experiment Station. Circular 347. [ Links ]
Kara, Z.; Arslan, D.; Guler, M. & Guler, S. (2015) - Inoculation of arbuscular mycorrhizal fungi and application of micronized calcite to alive plant: effects on some biochemical constituents of olive fruit and oil. Scientia Horticulturae, vol. 185, p. 219-227. https://doi.org/10.1016/j.scienta.2015.02.001 [ Links ]
Kitao, M. & Lei, T. (2007) - Circumvention of over-excitation of PSII by maintaining electron transport rate in leaves of four cotton genotypes developed under long-term drought. Plant Biology, vol. 9, n. 1, p. 69-76. https://doi.org/10.1055/s-2006-924280 [ Links ]
Kramer, P. & Boyer, J. (1995) - Evolution and agriculture. In: Kramer, P. & Boyer, J (Eds.) - Water relations of plant and soils. San Diego: Academic, p. 377-404. [ Links ]
Lynch, J.P. (2013) - Steep, cheap and deep: an ideotype to optimize water and N acquisition by maize root systems. Annals of Botany, vol. 112, n. 2, p. 347-357. https://doi.org/10.1093/aob/mcs293 [ Links ]
Miranda, D.; Fischer, G.; & Ulrichs, C. (2011) - The influence of arbuscular mycorrhizal colonization on the growth parameters of cape gooseberry (Physalis peruviana L.) plants grown in a saline soil. Journal Soil Science and Plant Nutrition, vol. 11, n. 2, p. 18-30. https://doi.org/10.4067/S0718-95162011000200003 [ Links ]
Mirshad, P. & Puthur, J. (2017) - Drought tolerance of bioenergy grass Saccharum spontaneum L. enhanced by arbuscular mycorrhizae. Rhizosphere, vol. 3, n. 1, p. 1-8. https://doi.org/10.1016/j.rhisph.2016.09.00. [ Links ]
Mittler, R. (2017) - ROS are good. Trends in Plant Science, vol. 22, n. 1, p. 11-19. https://doi.org/10.1016/j.tplants.2016.08.002 [ Links ]
Oliveira, J.W.; Bastos, M. & Cuquel, F.L. (2017) - Maintenance pruning in physalis commercial production. Bragantina, vol. 76, n. 2, p. 214-219. http://dx.doi.org/10.1590/1678-4499.128 [ Links ]
Ortiz, N.; Armada, E.; Duque, E.; Roldan, A. & Azcon, R. (2015) - Contribution of arbuscular mycorrhizal fungi and/or bacteria to enhancing plant drought tolerance under soil conditions: Effectiveness of autochthonous or allochthonous strains. Journal of Plant Physiology, vol. 174, p. 87-96. https://doi.org/10.1016/j.jplph.2014.08.019. [ Links ]
Pabon, J. (2012) - Cambio climático en Colombia: Tendencias en la segunda mitad del siglo XX y escenarios posibles para el siglo XXI. Revista de la Academia Colombiana de Ciencias Exactas, Físicas y Naturales, vol. 36, n. 139, p. 261-278. [ Links ] .
PRICC (2014) - Identificación de medidas y formulación de proyectos de mitigación y adaptación a la variabilidad y al cambio climático en la región capital Bogotá- Cundinamarca. Plan Regional Integral de Cambio Climático para Bogotá Cundinamarca. 253 p. [ Links ]
Qiu, Z.; Wang, L. & Zhou, Q. (2012) - Effects of bisphenol A on growth, photosynthesis and chlorophyll fluorescence in above ground organs of soybean seedlings. Chemosphere, vol. 90, n. 3, p. 1274-1280. https://doi.org/10.1016/j.chemosphere.2012.09.085 [ Links ]
Quiroga, G.; Erice, G.; Aroca, R.; Chaumont, F. & Ruiz-Lozano, J.M. (2017) - Enhanced drought stress tolerance by the arbuscular mycorrhizal symbiosis in a drought-sensitive maize cultivar is related to a broader and differential regulation of host plant aquaporins than in a drought-tolerant cultivar. Frontiers in Plant Science, vol. 8, art. 01056. https://doi.org/10.3389/fpls.2017.0105. [ Links ]
Ramadan, M.F. (2011) - Bioactive phytochemicals, nutritional value, and functional properties of Cape gooseberry (Physalis peruviana): an overview. Food Research International, vol. 44, n. 7, p. 1830-1836. https://doi.org/10.1016/j.foodres.2010.12.042 [ Links ]
Schonfeld, M; Johnson, R.; Carver, B. & Mornhinweg, W. (1988) - Water relations in winter wheat as drought resistance indicator. Crop Science, vol. 28, n. 3, p. 526-531. https://doi.org/10.2135/cropsci1988.0011183X002800030021x. [ Links ]
Yen, C.; Chiu, C.; Chang, F.; Chen, J.; Hwang, C.; Hseu, Y.; Yang, H.; Lee, A.; Tsai, M. & Guo, Z. (2010) - 4β-Hydroxiwithanolide E from Physalis peruviana (golden berry) inhibits growth of human lung cancer cells through DNA damage, apoptosis and G2/M arrest. BMC Cancer, vol. 10, n. 48, p. 2-8. https://doi.org/10.1186/1471-2407-10-46 [ Links ]
Yooyongwech, S.; Samphumphuang, T.; Tisarum, R. & Cha-Um, S. (2016) - Arbuscular mycorrhizal fungi (AMF) improved water deficit tolerance in two different sweet potato genotypes involves osmotic adjustments via soluble sugar and free proline. Scientia Horticulturae, vol. 198, p. 107-117. https://doi.org/10.1016/j.scienta.2015.11.002 [ Links ]
Zhao, R.; Guo, W.; Bi, N.; Guo, J.; Wang, L.; Zhao, J. & Zhang, J. (2015) - Arbuscular mycorrhizal fungi affect the growth, nutrient uptake and water status of maize (Zea mays L.) grown in two types of coal mine spoils under drought stress. Applied Soil Ecology, vol. 88, p. 41-49. https://doi.org/10.1016/j.apsoil.2014.11.016 [ Links ]
Zhou, Q.; Ravnkov, S.; Jiang, D. & Wollenweber, B. (2015) - Changes in carbon and nitrogen allocation, growth and grain yield induced by arbuscular mycorrhizal fungi in wheat (Triticum aestivum L.) subjected to a period of water deficit. Plant Growth Regulation, vol. 75, n. 3, p. 751-760. https://doi.org/10.1007/s10725-014-9977-x [ Links ]
Received/recebido: 2018.05.05
Received in revised form/recebido em versão revista: 2018.08.21
Accepted/aceite: 2018.10.05