Introduction
Although the scientific community of earth sciences has long been interested by the mechanisms governing both internal and external geodynamic processes, Geohazards is a recent scientific concept within the realm of Natural Hazard and Risk studies. This term encompasses a wide array of geomorphological, geological, and environmental processes, phenomena, and conditions, which have the capacity to result in a range of harmful consequences, including loss of life, injuries, adverse health impacts, damage to properties, disruption of livelihoods and essential services, economic and social disruptions, as well as environmental damage (Komac & Zorn, 2013). Geohazards exhibit varying temporal characteristics, as they can either rapidly emerge within seconds or minutes due to the driving processes or evolve gradually over extended periods of tens, hundreds, or even thousands of years before reaching a critical point where they become hazardous (Culshaw, 2018). Geohazards are found in most natural and artificial systems of the world and include subaerial and submarine processes, such as earthquakes, volcanic eruptions, tsunami, coastal erosion, landslides, sinkholes, floods, and soil erosion.
This work addresses the state of the art of geohazards in Portugal, including the Madeira and Azores. The article is organized into four sections, in addition to the introductory section. Section 2 highlights the pioneers in approaching geohazards in Portugal, whereas a bibliometric analysis based on the Scopus database is presented in Section 3. Section 4 addresses the comprehensive spatial and temporal incidence of geohazards in Portugal, based on extensive published scientific work. The final remarks are summarized in Section 5.
Pioneers in studying geohazards in Portugal
Among the first contributions towards the study of geohazards in Portugal, two geographers stand out: Fernando Rebelo from the University of Coimbra and António de Brum Ferreira from the University of Lisbon.
In 1967, Rebelo carried out a pioneering study in Portugal on the dynamic of slopes along the valley of the Dueça river. In this work, Rebelo (1967) identified soil erosion and landslides as the dominant processes in current morphogenesis and related them to the lithology, tectonics, slope gradient, slope profile, and climatic conditions. In a latter work, based on observations made in a limestone area near Condeixa, Rebelo (1982) discussed types of gully erosion and pointed out the lithology, the slope gradient, the rainfall characteristics (intensity and frequency) and the anthropogenic action (namely the destruction of vegetation cover) as determining factors for gully development.
In the first article addressing landslides in the Lisbon region, Ferreira (1984) discussed the geomorphological conditions favourable to the current slope dynamics. Additionally, Ferreira (1984) mapped landslides that occurred in Calhandriz and Adanaia in February 1979, while analysing the climatic and hydrological factors that triggered these landslides. Ferreira was a pioneer in attempting to establish relationships between precipitation and landslides in Portugal. This author concluded that a strong association exists between the type of landslides and precipitation. According to Ferreira (1984), small soil slips and rockfalls are induced by torrential showers concentrated in a few hours and are associated with convective depressions, whereas deep-seated landslides are induced by long lasting rainy periods that occur during the cold season.
A bibliometric reading
A comprehensive bibliometric analysis focusing on geohazards in Portugal was conducted using data from the up-to-date Scopus database (scopus.com), current as of September 2023. The methodology involved two phases. In the initial phase, an extensive search was conducted, encompassing titles, abstracts, and keywords. This search involved the combination of terms like 'Portugal,' 'Madeira,' or 'Azores' with a range of geohazard-related terms, such as hazard, earthquake, volcano, tsunami, coastal erosion, beach erosion, landslide, debris flow, sinkhole, flood, soil erosion, and gully erosion. The resulting 36 distinct searches yielded a total of 1838 references, which were organized in a dedicated database. Subsequently, a refinement process was implemented in the second phase, involving a thorough examination of the content of each identified article. This analysis enabled the exclusion of 701 references that did not align with the research objectives or criteria.
The final set of 1137 curated references were then saved within the Scopus platform and subjected to in-depth analysis using the descriptive analysis tool provided by Scopus. Additionally, these references were exported as a CSV file containing the complete information relating citation, bibliography, abstract and keywords. To further explore and visualize the bibliometric data, the CSV file was employed as input for the VOSviewer software (version 1.6.19). This software facilitated the analysis of clusters and the creation of network-based bibliometric maps (Van Eck & Waltman, 2010, 2023).
The descriptive statistics of publications addressing geohazards in Portugal up to September 2023 are summarized in figure 1. Although the first indexed work was published in 1970, the annual number of publications was minimal until the end of the 1980s. The largest increase in the number of published works occurred since the beginning of the 21st century, with approximately 72% of the total works being published after 2010 (fig. 1A).
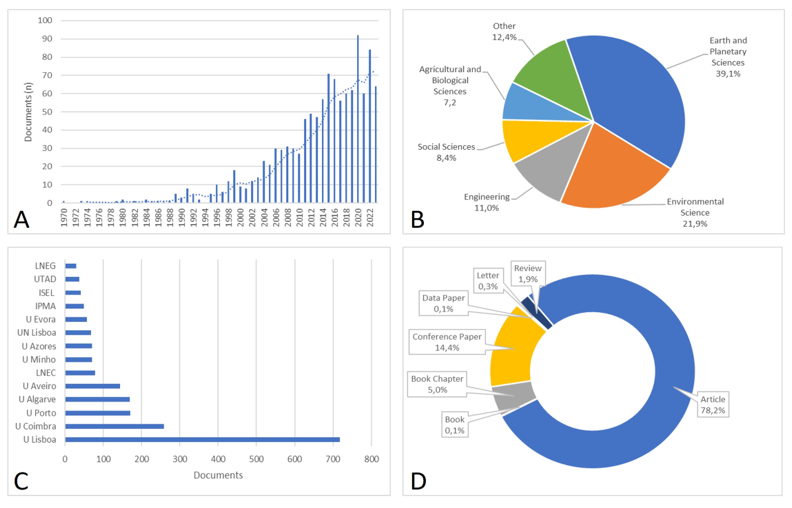
Fig. 1 Descriptive statistics of publications on Geohazards in Portugal indexed by Scopus (September 2023). A - Number of documents (dashed line - 5-year moving average); B - Documents by subject area; C - Documents by affiliation; D - Documents by type.
Most research on geohazards has been published in the fields of Earth and Planetary Sciences (39%) and Environmental Science (22%) (fig. 1B). Additionally, contributions from Engineering, Social Science, and Agricultural and Biological Sciences make up 11%, 8%, and 7% of the total publications, respectively.
In Portugal, articles have been the predominant type of publication for geohazard topics, comprising 78% of the total publications, followed by Conference Papers at 14% (fig. 1D). In contrast, Books and Book Chapters together account for only 5% of the total publications.
The total number of affiliations exceeds the number of documents, primarily because many articles have multiple affiliations. figure 1C provides an overview of the document distribution among the 14 Portuguese academic and technical institutions more effective in publishing on geohazard topics, representing 70% of the total. The remaining 30% primarily involves foreign institutions. The University of Lisbon leads with over 700 documents, followed by the University of Coimbra with 259 documents. Following closely are the universities of Porto (171), Algarve (170), and Aveiro (144).
The clustering of citation sources is depicted in figure 2, utilizing data from 1137 published articles indexed in the Scopus database. The degree of association between items is established by the frequency of their co-occurrence in documents. Generally, the closer two journals are positioned within the cluster, the more pronounced their interrelation. In addition, the most robust co-citation connections amng journals are visually indicated by connecting lines.
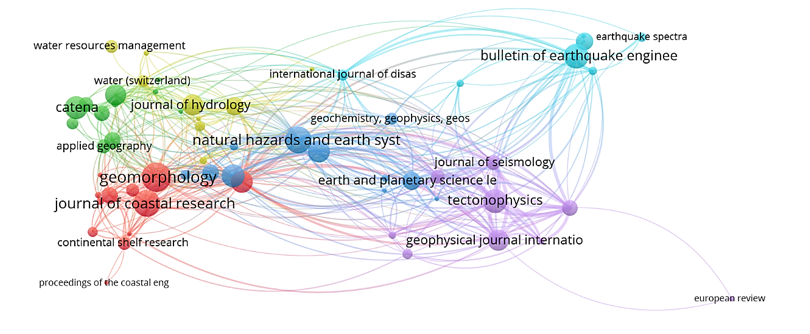
Fig. 2 Citation sources clustering based on 1137 published articles indexed in the Scopus database. The minimum number of documents per source was set as 5.
In the centre of the picture, two journals that specialize in publishing research on hazards stand out: Natural Hazards and Earth System Science and Natural Hazard. This central cluster is surrounded by five other clusters that are associated with specific geohazards: landslides and coastal erosion (e.g., Geomorphology and Journal of Coastal Research), soil erosion (e.g., Catena and Water), and floods (e.g., Journal of Hydrology). It is noteworthy that earthquakes are linked to two clusters, one connected to engineering (e.g., Bulletin of Earthquake Engineering) and the other aligned with geosciences (e.g., Tectonophysics and Geophysical Journal International). These geohazards, along with 'tsunami,' are prominently featured in the clustering of co-occurring author keywords (fig. 3). This clustering also highlights several analytical methods, such as numerical modelling, fragility curves, and damage scenarios, along with elements of the conceptual risk model, including susceptibility, hazard, exposure, and vulnerability components.
The analysis of co-authorship is illustrated in figure 4, which emphasizes eight major thematic clusters: landslides and floods, coastal erosion, estuarine floods, soil erosion, tsunamis, volcanoes, and earthquakes, the later examined from both engineering and geosciences perspectives. Soil erosion is primarily linked to researchers from the University of Aveiro, while volcanoes are predominantly associated with researchers from the University of Azores. Within the clusters of landslides and floods, tsunami, and earthquakes (geosciences), researchers from the University of Lisbon hold a dominant position, along with researchers from the University of Évora in the case of earthquakes. The cluster related to earthquakes (engineering) is mainly led by researchers from the University of Minho and the University of Lisbon. Coastal erosion has been primarily studied by researchers from the Universities of Algarve and Lisbon, while estuarine flooding has been mainly investigated by researchers from LNEC and the University of Aveiro.
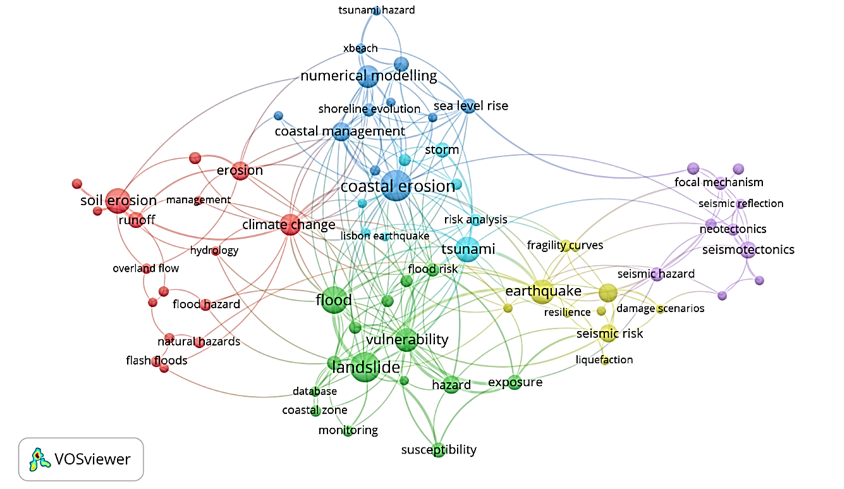
Fig. 3 Co-occurrence author keywords. A thesaurus was used to eliminate plural words. Terms of regional toponomy were removed.
Spatial and temporal incidence of geohazards
Earthquakes and volcanic eruptions
The tectonic environment plays a significant role in neotectonic and seismic activity in Portugal (Cabral, 1995). Mainland Portugal is situated north of the plate boundary where the Nubia plate to the south meets the Eurasian plate to the north. Locally, the convergence between these two plates occurs obliquely at a rate of approximately 5mm year-1 (Fernandes et al., 2007). The primary adjustment of tectonic convergence occurs predominantly in the offshore plate boundary region, located south of mainland Portugal (Carrilho et al., 2021). This area is the transitional zone between the subducted Gibraltar slab and Mediterranean back-arc region to the east and the long strike-slip Gloria fault to the west, which connects to the Azorean triple junction, through the Terceira Ridge Leaky Transform (Baptista, 1998; Bezzeghoud et al., 2011; Cabral, 2012; Cunha et al., 2012; Grandin et al., 2007; Silva et al., 2011; Zitellini et al., 2009).
In a recent work, Carrilho et al. (2021) summarized the seismicity of the Azores-Madeira-Iberian region, taking into account the earthquakes recorded instrumentally since 1961 and the historical earthquakes retrieved from the Seismic Harmonization in Europe (SHARE) European Earthquake Catalog (SHEEC), with magnitudes larger than 5.5 (Stucchi et al., 2013). Along the plate boundary known as the Azores-Gibraltar plate boundary, from west to east, multiple low-magnitude earthquakes have been recorded in the Terceira Ridge Leaky Transform (Baptista, 1998).
Higher-magnitude earthquakes have been linked to the Glory Fault, even though their epicentres are spatially dispersed (Baptista, 1998). The boundary between the Nubia and Eurasian plates is vaguely defined eastward from the Glory Fault (Bezzeghoud et al., 2011; Cabral, 2012; Cunha et al., 2012; Zitellini et al., 2009), and frequent earthquakes occur in the offshore zone southwest and south of mainland Portugal.
Evidence pointing to a subduction zone along the southwestern Iberian margin was summarized by Duarte et al. (2013), supporting the hypothesis of a transition from a passive to an active margin in the boundary domain, which was proposed earlier by Cabral and Ribeiro (1989). According to Cabral (1995), this peculiar geodynamic context could explain the occurrence of exceptionally high-magnitude earthquakes as the one happened in 1755.
The work by Carrilho et al. (2021) also summarizes the surface projection of potentially active faults onshore and offshore in the Azores-Madeira-Iberian region, following proposals of Cabral (2012), Basili et al. (2013), Duarte et al. (2013) and Vilanova et al. (2014). Cabral (2012) distinguishes two main groups of active faults in mainland Portugal, which are additional sources of seismic activity: N-S to NNE-SSW left-lateral strike-slip faults (such as the Manteigas-Vilariça-Bragança and the Penacova-Régua-Verin Faults), and E-W to NE-SW reverse faults (such as the Vidigueira-Moura Fault, the south-verging Ponsul Fault, and the north-verging Seia-Lousã Fault). Furthermore, the Lower Tagus Valley constitutes a significant intraplate seismic zone in Portugal and has been the origin of several M 6-7 earthquakes, such as those occurring in 1344, 1531, and 1909 (Cabral et al., 2013). The Lower Tagus Valley is within the Tagus Cenozoic Basin that encompasses up to 2000 m of sediments dating from the Paleogene up to the Quaternary. The location and kinematics of the Lower Tagus Valley Faut Zone have been subject to debate. While Cabral et al. (2013) interpreted this structure as a blind reverse fault, Vilanova and Fonseca (2004) advocate for strike-slip kinematics. According to Cabral et al. (2013), previously existing normal faults have been transformed into southeast-verging reverse faults, along the NW contact between the Tagus Cenozoic Basin and the Lusitanian Basin. The Vila Franca de Xira fault is an example of this tectonic inversion, being a reliable candidate for the source of the 1531 earthquake (Cabral et al., 2013). Despite the recognized seismic activity, the consistent relationship between the earthquakes and the faults recognized at the surface in the Lower Tagus Valley is missing, which is explained by the low slip rates estimated for regional active faults (0.05-0.1mm year-1), together with the thick quaternary sediment cover that hinder the evidence of surface rupturing (Cabral et al., 2013). Also, the 1909 Benavente earthquake occurred in this area, without apparent correspondence with any recognized active structure on the surface, implying that the seismic event may have originated along a blind thrust fault underneath the Cenozoic detrital formations. The focal mechanism analysis revealed that the source of the seismic event is likely an ENE reverse fault (Stich et al, 2005), which eventually connects the Vila Franca de Xira and Azambuja faults (Cabral et al., 2004, 2013; Carvalho, 2003).
According to Canora et al. (2021), the Lower Tagus Valley Fault exhibits two almost parallel strands running along both sides of the Tagus alluvial plain. Based on geomorphological, palaeoseismological and seismic reflection data, the Eastern Lower Tagus Valley Fault Zone was characterized as a seismic area able to generate earthquakes up to 7.3Mw maximum magnitude with slip rate of 0.14-0.24mm year-1, and 1.7ky recurrence interval for surface rupture (Canora et al., 2015).
Mainland Portugal experiences a concentration of earthquakes along two specific coastal zones: the southern Algarve coastal zone and the west coastal zone, primarily between Sagres and the Nazaré Canyon. The spatial distribution of maximum seismic intensity was updated in 1996 (Agência Portuguesa do Ambiente [APA], 2010) using historical and instrumental data, revealing that the highest values have been observed in the south-west region of Portugal, particularly in the Algarve, Lisbon, and the Lower Tagus Valley, where the seismic intensity reached levels IX and X on the Modified Mercalli scale. In a recent work, Teves-Costa et al. (2019) proposed a novel detailed maximum intensity map for Portugal mainland, based on the revision and compilation of the available macroseismic information containing 5544 intensity data points.
The seismic activity in Portugal has been characterized by a few high-magnitude events. Since 63 BC there have been 59 earthquakes with body-wave magnitude (Mb) equal to or greater than 6. Table I lists 16 highly destructive earthquakes that affected mainland Portugal, with particular emphasis on the devastating 1755 Lisbon earthquake.
Table I Major earthquakes that affected the Portuguese mainland (Mb-body-wave magnitude; Mw-moment magnitude, K - Imamura Papadopoulos scale).
ear | Month | Day | Name | Source location | Magnitude Mb/Mw | Tsunami intensity (K) |
---|---|---|---|---|---|---|
1969 | 02 | 28 | Horseshoe EQ | Horseshoe Abyssal Plain | 7.5/- | III |
1964 | 03 | 15 | Guadalquivir Bank EQ | Gulf of Cadiz | 6.2/- | |
1909 | 04 | 23 | Benavente EQ | Lower Tagus Valley | -/6.0 | |
1858 | 11 | 11 | Setúbal EQ | South of Setúbal | 6.8/- | |
1856 | 01 | 12 | Sotavento EQ | SW Iberian margin | 6.0/- | |
1761 | 03 | 31 | North Atlantic | Gloria Fault Zone | 7.5/- | VI |
1755 | 11 | 01 | Lisbon EQ | SW Iberian margin | -/8.5 - 8.7 | XI |
1722 | 12 | 27 | Tavira EQ | Offshore Algarve | 6.5/- | VI |
1719 | 03 | 06 | Portimão EQ | Offshore (?) Algarve | 7.0/- | |
1531 | 01 | 26 | Vila Franca de Xira EQ | Lower Tagus Valley | 7.1/- | VII |
1512 | 01 | 28 | Lisbon EQ | Lower Tagus Valley | 6.3/- | |
1356 | 08 | 24 | North Atlantic | Gloria Fault Zone | 7.5/- | |
1353 | Silves EQ | Offshore Algarve | 6.0/- | |||
1344 | Benavente EQ | Lower Tagus Valley | 6.0/- | |||
382 | Cape S. Vicente EQ | SW Iberian margin | 7.5/- | VI | ||
63 BC | Portugal and Galicia EQ | W Iberian margin | 8.5/- | VII |
Source: based on Delgado et al., 2011; Gràcia et al., 2010; Martins and Mendes-Victor 2001; Oliveira 1986; Teves-Costa et al., 1999); Tsunami data is based on Baptista and Miranda (2009)
The Lisbon earthquake occurred on the 1st of November 1755 stands as the most powerful seismic occurrence ever recorded along the European Atlantic shores. The level of destruction was very high across the coastal zone of Portugal, Spain, and Morocco, primarily due to the earthquake-triggered tsunami waves (Baptista et al., 2003, Martínez Solares and Lopez Arroyo, 2004).
Three seismic sources have been proposed for the Lisbon earthquake: the Gorringe Bank, the Marquês de Pombal Fault and the Gulf of Cadiz (Zitellini et al., 2001). However, when considered individually, these seismic sources are deemed insufficient in size to account for the extensive seismic effects observed during the earthquake, as indicated by the recorded intensities.
In a recent paper, Silva et al. (2023) conducted a macroseismic analysis of the AD 1755 Lisbon Earthquake-Tsunami event, combining intensity data derived from the EMS-98 scale and the ESI-07 scale, which focuses on environmental damage. More than 900 records of secondary earthquake environmental effects were used for Spain and Portugal. These authors concluded that the occurrence of the Lisbon earthquake can only be adequately explained when considering a combination of the abovementioned three seismic sources. These sources are likely associated with shallow subduction or lithospheric delamination beneath southwestern Iberia and the Gulf of Cadiz. The analysis suggests that the offshore rupture extends along the NNE-SSW direction, spanning approximately 350-360km and resulting in an event magnitude 8.6Mw estimated from empirical relationships (Silva et al., 2023).
Using an alternative approach, Fonseca (2020) estimated that a significant portion of the complete macroseismic dataset (96.4%) of the 1755 Lisbon earthquake can be accounted for by a single offshore source located in the western Gulf of Cadiz with magnitude 7.7 ± 0.5. The remaining 3.6% of the data, which includes intensities MMI from VIII to X, have been tentatively attributed to secondary onshore ruptures, eventually along the Lower Tagus Valley (Vilanova et al., 2003).
The seismic hazard is negligible in Madeira and Porto Santo. On contrary, the Azores archipelago is very active seismically, in particular the islands belonging to the central and eastern groups. Gaspar et al. (2015) listed 31 destructive earthquakes that were registered in the Azores, since the settlement of the archipelago in the fifteenth century. Major earthquakes occurred in historical times have reached magnitudes >7 and often triggered landslides and tsunamis. The first map of observed seismic intensity for the Azores islands was provided by Fontiela et al. (2017), showing intensities (MMI) above VII in the central and eastern groups. The highest values (MMI = XI) were reported for São Miguel island (eastern group) and São Jorge island (central group). The most recent destructive earthquakes that hit Azores were the Terceira earthquake, occurred on the 1st of January 1980 (Mb 7.2) and the Faial Earthquake, occurred on the 9th of July 1998 (Mb 5.6).
Volcanic activity is currently confined to the Azores archipelago. Gaspar et al. (2015) documented 28 volcanic eruptions that have been recorded in the Azores since the fifteenth century. These eruptions encompass both submarine and subaerial events, with the latter ranging from Hawaiian to sub-Plinian types, exhibiting hydromagmatic characteristics in some instances (Gaspar et al., 2015).
Tsunami
Tsunamis may be generated by strong earthquakes with epicentre in the ocean floor, but also by volcanic eruptions and submarine landslides. Baptista and Miranda (2009) compiled a list of 15 tsunami events that had huge impacts along the Portuguese coastal zone. Out of the 15 events, seven were caused by earthquakes with magnitude higher than 6.5 (table I). Four of these tsunamis are considered regional or Atlantic-wide in scale: 63 BC, 382, 1755 and 1761. The tsunamis generated by these earthquakes led to the inundation of large areas along the Portuguese coast and caused significant damage. Among the recorded events, the 1755 tsunami had a devastating impact, resulting in a high number of fatalities, namely on the Lisbon city. Moreover, three of these tsunamis (63 BC, 382, and 1755) were noted to have caused morphological changes to the Portuguese coastal landscape (Baptista and Miranda 2009). The tsunami catalogue also includes a tsunami triggered by a large submarine landslide (1929 Grand Banks event) and a tsunami triggered by a rockfall affecting a coastal cliff in the Madeira Island that resulted in 19 fatalities (1930 Cabo Girão event; Omira et al., 2022).
Recently, 16 site locations containing tsunami deposits along the Portuguese coastal zone were listed by Costa et al. (2022), which includes information on age, generation mechanism and geomorphological setting. In addition, tsunami deposits offshore generated by the 1755 Lisbon tsunami and a ca. 3600 cal yr BP tsunami event have been studied on the Algarve shelf using sedimentology, geochemistry, hydroacoustics and radiocarbon dating (Feist et al., 2023).
The modelling of the 1755 tsunami propagation has been attempted using the shallow water equations and different earthquake fault parameters and location: the Gorringe Bank (e.g. Santos & Koshimura, 2015a); the continental shelf located between the Gorringe Bank and the Iberian coast (Baptista et al., 1998), the Horseshoe Fault (Baptista et al., 2011), and the Gulf of Cadiz (Gutscher et al., 2006).
A first criterion to assess tsunami hazard in the Portuguese coastal zone was proposed by Santos et al. (2011), aggregating the water level height and travel times of the tsunami waves for a scenario comparable to the 1755 event. More recently, Santos and Koshimura (2015b) improved the former criterion and concluded that the tsunami hazard is highest along the coastal faced to the West, stretching from Sagres to the Cabo da Roca.
Omira et al. (2015) presented the first probabilistic earthquake-triggered tsunami hazard assessment for the Northeast Atlantic region. The methodology combines the probabilistic seismic hazard assessment, tsunami numerical modelling, and statistical approaches, and considering three major tsunami source areas: the Southwest Iberian Margin, the Gloria Fault, and the Caribbean. The obtained hazard levels point out the southern Portuguese coast, the NW cost of Morocco and the SW coast of Spain as the most hazardous zones along the NE Atlantic. In addition, most tsunami hazard in the Azores originates from far-earthquake sources located in the Caribbean region (Omira et al., 2015).
Coastal erosion
The coastal zone of mainland Portugal stretches for 830km and comprises three primary geomorphologic features: Beaches, Coastal Cliffs, and Low Rocky Coasts. The geomorphologic characterization of the Portuguese coastal zone is beyond the scope of this work. Further information about this subject can be found in Abecasis (1997), Andrade et al. (2002), and Ferreira and Matias (2013), and references therein.
Since the late nineteenth century, Portugal has been facing with the issue of coastal erosion due to the regressive coastline trend. In response, the first coastal engineering protection were made in the early twentieth century to safeguard the village of Espinho, situated south of Oporto. However, such coastal defences proved ineffective, and over a 1000m stretch, houses and streets were destroyed (Valle, 1989).
Existing coastal hazards in Portugal are associated to slope instability on rocky coastal cliffs, as well as beach erosion along low-lying sandy coasts (Zêzere, 2020). Coastal cliffs located from Nazaré to Peniche are commonly affected by rockfalls and rockslides disrupting stratified rocks (limestone, marl, sandstone, clay) dated from the Jurassic and the Cretaceous. In the Algarve south coast, around Alvor - Olhos de Água, cliffs cutting soft limestone and sandstone of Miocene age are also unstable, being affected by rockfall, gully erosion and sinkhole collapse. Nowadays, the accelerated retreat of sandy beaches is the major geomorphological threat along the Portuguese coastal zone. Ponte Lira et al. (2016) presented the first long-term coastline evolution of low-lying sandy coasts in mainland Portugal. These authors quantified the coastline evolution by mapping and comparing the foredune toe within sandy beach-dune systems, observed in aerial photos dated from 1958 and 2010. Sandy beach-dune systems account for 40% of the total Portuguese mainland coastal zone and depict an overall erosional tendency with a mean change rate of −0.24 m year−1. The highest erosion rates were found in the coastal stretches of Espinho-Torreira (up to −4.5 m year−1), Costa Nova-Praia de Mira (up to −7.4 m year−1), Cova da Gala-Leirosa (up to −3.8 m year−1), and Cova do Vapor-Costa da Caparica (up to −4.6 m year−1). Moreover, Lira et al. (2016) concluded that zones of intense coastal erosion are associated with human-induced deficit in the sediment budget, namely along the coastal stretch between the Minho river and Nazaré, as well as in the Costa da Caparica spot, where major engineering works have interfered with the coastal system.
Recently, Tavares et al. (2021) made available a comprehensive database documenting coastal flood events that occurred along the Portuguese coastal zone between 1980 and 2018. This extensive database was compiled from national and regional newspapers, encompassing 650 recorded occurrences and detailing 1708 associated impacts on individuals, infrastructure, public spaces, and the natural environment. Notably, the municipalities of Ovar, Almada, Porto, Ílhavo, and Vila Nova de Gaia emerge as having experienced the highest number of occurrences.
The accelerated erosion along the Portuguese coastal zone has been driven by four major factors (Dias, 1993; Ramos-Pereira, 2004; Teixeira, 2014):
The sea level rise (SLR) resulting from the oceanic thermal expansion. Analysis of the long-term tide gauge data at Cascais reveals an average sea level rise of 1.9mm year-1 between 1920 and 2000, which increased to 3.6mm year-1 in the first decade of the twenty-first century (Antunes, 2011). The increasing rate of SLR observed in Cascais is probably related to climate change. Recently, Antunes (2019) projected for this tide gauge a mean SLR of 1.14m for the end of the 21st century, despite the wide range of 95% of probability between 0.39m and 1.89m.
The drop of sediment supply since the middle of the 20th century. Since the 1950s, the Portuguese coastal zone has experienced a decline in sediment supply, primarily attributed to human activities like dredging and inert extraction along rivers and estuaries. In addition, the construction of dams along the main Iberian rivers since the mid-twentieth century has also played a key role in reducing the influx of continental sediments (Dias, 1993; Grupo de Trabalho para o Litoral [GTL], 2014). It was estimated that dams currently retain over 80% of the sand volume that was previously transported by rivers to the coastal zone (Dias, 1993; Valle, 2014). Consequently, there is now a shortage of sand available for redistribution by the longshore drift, along the Portuguese west coastal zone.
The extensive development along the coastal zone. Over the years, there has been substantial urbanization and the development of harbours, industrial facilities, and tourist establishments, exerting pressure on the coastal environment. This rapid development has disrupted the coastal biophysical systems, being responsible for the disappearance of critical beach-dune systems (Ramos-Pereira, 2004).
The deployment of extensive coastal engineering works, which have generated undesirable effects on the coastal areas where they are implemented. The rigid nature of these engineering structures contrasts sharply with the high dynamics of the shore zone leading to disturbances within the coastal system (Dias, 1993). As a rule, harbour structures create artificial barriers that disrupt the natural circulation of sediments carried by the longshore drift. Similarly, engineered coastal groins also have a comparable impact, causing sediment accumulation updrift of the groin, while accelerating erosion downdrift. These adverse effects compromise the balance of sediment transport along the coast.
Landslides
Landslides occurred in Portugal have been triggered by earthquakes or rainfall. The deadliest landslides documented in Portugal were debris flows that destroyed Vila Franca do Campo (S. Miguel island, Azores) on the 22 October 1522. The debris flows were triggered by a strong earthquake (estimated magnitude 5.7−6.7) with epicentre located 14km NW of Vila Franca do Campo (Carmo et al., 2013; Gerrard et al., 2021; Marques et al., 2009). These landslides originated in the hills above Vila Franca do Campo, followed pre-existing fluvial channels, and spread out over the village and entering into the ocean (Marques et al., 2009). An estimated 3000 to 5000 lives were lost in Vila Franca do Campo, which was the most important settlement on the island at that time (Gerrard et al., 2021). Marques et al. (2009) mapped the field evidence of the debris flows deposits and modelled the flow propagation using a gravity flow model based on Monte-Carlo algorithm. The debris flow deposits covered 4.5km2 and the volume of the affected material was estimated as 6.75x10m6.
More recently, on 31 October 1997, more than 1000 shallow slides were triggered by a short, very intense rainfall event that hit the eastern part of S. Miguel island. Some of these slides evolved into debris flows, and one of them generated 29 dead people in the small village of Ribeira Quente (Valadão et al., 2002). The occurrence of rainfall-triggered landslides is also common in the Madeira island, where the combined landslide - flash flood event of 20 February 2010 was responsible for 45 casualties and 6 missed people (Fragoso et al., 2012; Lira et al., 2013; Lourenço & Rodrigues, 2015).
Vaz and Zêzere (2016) listed 28 earthquake-triggered landslides that occurred in mainland Portugal between 382 and 1969. These landsides were triggered by 10 seismic events with magnitude ranging from 4 to 8.7 and were distributed along the central and southern parts of Portugal. The registered slope movements were disrupted landslides affecting jointed rocks together with rockfalls, which took place during the 1531, 1755, 1909 and 1969 earthquakes (Vaz & Zêzere, 2016).
Landslides triggered by rainfall in Portugal’s mainland between 1865 and 2010 were summarized in the DISASTER database (Zêzere et al., 2014). More recently, this database was updated up to 2015 (Pereira, et al., 2017; Zêzere et al., 2020). The updated DISASTER database includes 302 damaging landslides triggered by rainfall that are distributed along the rainiest northern and central parts of Portugal, where the energy of the relief is highest.
Landslides have been also inventoried in the offshore zone west and southwest of Iberia, where more than fifteen hundred submarine landslides were identified and included into a database (Gamboa et al., 2021).
The typical landslide activity affecting the three major geologic-geomorphologic units that are found in mainland Portugal was summarized by Zêzere (2020) based on previous work by Rodrigues and Coelho (1989) and Ferreira and Zêzere (1997).
The Hercynian Massif primarily comprises granite and metasediments, with schists and greywackes being prevalent in the latter category. In the northern region, granite predominate, while metasediments prevail in the central and southern parts of Portugal. Granite experiences rockfalls on steep valley slopes, namely in highly fractured zones, whereas the residual soils resulting from the weathering of granite are affected by debris flows. Metasediments like schists and shales often experience shallow planar slides affecting the regolith and slope deposits covering the mountain slopes, in the central and northern regions of Portugal. Slip surfaces develop along discontinuities within the schists and shales, such as layering, foliation, and fracture planes, especially on moderate slopes (10-15°). In higher slopes, the planar shallow slides can transform into rapid debris flows and mudflows with devastating consequences. An illustrative example of such events occurred along the Douro valley in January 2001 (Pereira et al., 2014).
Landslides in the western and southern Meso-Cenozoic borderlands primarily result from factors like lithology, geological structure, and hydrogeological conditions. In the Algarve, landslides are more frequent over the Triassic-Hettangian evaporitic complex, along the peripheral depression that borders the Hercynian Massif. In the western Meso-Cenozoic borderland, three clay-rich lithologic units experience rotational slides, translational slides, and complex slope movements (Ferreira & Zêzere, 1997; Rodrigues & Coelho, 1989): the upper Jurassic marl and marly limestone, in the area between Lisbon and the Montejunto mountain; the upper Cretaceous marl and limestone, in Nazaré - Leiria − Vila Nova de Ourém and Lisbon - Cascais − Ericeira; and the upper Cretaceous marl, clay, sand, and sandstone, in Pombal - Condeixa - Soure − Aveiro.
In the Tagus and Sado Cenozoic Basin landslides commonly take the form of shallow translational slides, mudslides, topples, and rockfalls. The occurrence of these landslides is closely linked to the existence of over consolidated Miocene clays in the Lisbon region and Miocene detrital formations found in the Santarém region.
Extensive work on rainfall thresholds for landslide activity in Portugal have showed that the critical rainfall conditions for slope failure vary substantially depending on the landslide type and are greatly affected by the local geological and geomorphological features (Ferreira et al., 2005; Marques et al., 2007; Quinta- Vaz et al., 2018; Zêzere & Rodrigues, 2002; Zêzere et al., 2005, 2008, 2015). In the case of rapid debris flows, they are usually initiated by extremely heavy rainfalls occurring over a short duration, typically within a few hours. Conversely, shallow translational soil slips are typically triggered by intense precipitation events spanning a range of 1 to 15 days. In contrast, deep-seated landslides, including rotational, translational, and complex types, are more closely associated with prolonged periods of nearly continuous rainfall, which can persist from several weeks to several months (Zêzere et al., 2015).
In the last two decades, landslide susceptibility has been assessed in Portugal at the basin and municipal scales, using heuristic (e.g. Gomes et al., 2005; Ramos et al., 2014; Santos, 2015), statistic (e.g. Cunha et al., 2017; Guillard & Zêzere, 2012; Melo & Zêzere, 2017; Pereira et al., 2012; Silva et al., 2018; Zêzere, 2002; Zêzere et al., 2004, 2017) and physically-based method (e.g. Marques et al., 2020; Melo et al., 2018; Oliveira et al., 2017; Riscado et al., 2012; Teixeira et al., 2015).
A landslide risk index was recently proposed for municipal land use planning in Portugal (Pereira et al., 2020), resulting from the crossing between landslide susceptibility, exposure, and physical vulnerability of buildings. The landslide susceptibility was extracted from a national landslide statistically based susceptibility model (Zêzere et al., 2018), which was based on a comprehensive landslide inventory containing 7387 landslides represented as polygons. These landslides were identified through a combination of photo-interpretation and fieldwork conducted across 14 test sites that were chosen to represent the diverse geology and geomorphology of the country. The Portuguese municipalities were clustered according to the landslide risk drivers (susceptibility, exposure, physical vulnerability), contributing to context-oriented strategies of landslide risk management (Pereira et al., 2020).
Floods
Floods and flash floods pose significant hazards and risks to Portugal. In a recent study that update the DISASTER database, Zêzere et al. (2020) examined 1658 hydrologic disaster cases that had human consequences in mainland Portugal between 1865 and 2015. These floods and flash floods were accountable for 1012 fatalities, averaging 6.8 deaths per year. Additionally, they led to the displacement of 14 061 people and left 40 365 individuals without home.
Hydrologic disasters were observed throughout the country, but certain areas experienced a higher concentration of flood cases. These critical areas include the Lisbon region and the Tagus valley, the Oporto region and the Douro valley, the Coimbra region, and the Mondego valley, as well as the Vouga River valley (Zêzere et al., 2014, 2020).
Floods are prevalent in the major rivers such as the Tagus, Douro, Mondego, Sado, and Guadiana rivers. On the other hand, flash floods primarily occur in smaller watersheds, notably within the Lisbon Metropolitan Area, as well as along the West and the Algarve regions.
Floods along major rivers develop gradually over time typically due to extended periods of rainfall during the Winter. The flood management techniques now rely on controlling dam discharges to avert most flooding incidents. However, dams can inadvertently exacerbate extreme floods when their reservoirs approach full capacity, requiring discharge during extended periods of heavy rain, as occurred during the Tagus flood in March 1978 (Daveau et al., 1978).
As a rule, flash floods generate risk levels higher than floods in major rivers, particularly when they strike highly urbanized areas (Zêzere, 2020). The flash flood hazard is related to the short concentration time within small catchments, coupled with the typical occurrence of high peak discharge together with a very high sediment load. Flash floods have been triggered by intense rainfall showers condensed into a few hours, mostly during the autumn and spring seasons.
Estuaries are sensitive zones highly prone to flooding, namely due to the ongoing sea level rise (SLR). A flood database for the Tagus estuary based on historical information was presented by Rilo et al. (2017). Guerreiro et al. (2015) evaluated the effect of SLR on the tidal propagation and circulation along the Tagus estuary, using field data to calibrate and validate a high-resolution hydrodynamic model. The authors concluded that future extreme water levels will be higher than the sum of present levels with the SLR. In a recent work, Lopes et al. (2022) evaluated future estuarine floods of three major rivers (Tejo, Sado and Mondego) together with the Ria de Aveiro and the Ria Formosa, integrating the computation of local extreme sea levels with high resolution numerical modelling. The obtained results showed the control of extreme sea levels and the estuarine geomorphology over the inundation extent. Moreover, the authors concluded that the extent of future estuarine inundation will be smaller than that obtained by the bathtub approach (e.g. Antunes et al., 2019), due to the attenuation of long wave height along the estuaries (Lopes et al., 2022).
The top 10 hydrogeomorphological disaster events occurred in mainland Portugal in the period 1865 - 2015 are summarized in table II. These events were marked by the prevalence of floods, flash floods, urban flooding, and landslides, typically covering large areas and impacting multiple districts in Portugal. Remarkably, more than half of all fatalities resulting from hydrologic disasters in mainland Portugal during the last 150 years stemmed from a single flash flood event that occurred in the Lisbon region on 25-26 November 1967, resulting in at least 522 deaths (Zêzere et al., 2014). The Lisbon region flash flood occurred in 1967 was characterized by Trigo et al. (2016) that highlighted the Póvoa sub-basin and the Quintas village as major flood disaster spots.
Table II Major disaster events of hydrogeomorphological origin in mainland Portugal in the period 1865-2015.
Date | Event type | Affected Districts | Fatalities | Injured people | Evacuated people | Displaced people |
---|---|---|---|---|---|---|
2001 26-27 Jan | F + L | 1, 3, 6, 9, 10, 13, 17, 18 | 6 | 5 | 402 | 570 |
1997 2-9 Nov | FF + UF | 2, 8, 14, 15 | 11 | 22 | 141 | 134 |
1983 18-19 Nov | FF + UF + L | 11, 14 | 18 | 0 | 255 | 3 239 |
1979 5-16 Feb | F + FF + L | 5, 6, 11, 13, 14, 17 | 8 | 3 | 4244 | 14 322 |
1967 25-26 Nov | FF + UF | 11, 15 | 522 | 330 | 304 | 885 |
1941 15-17 Feb | F + FF | 11, 15 | 33 | 0 | 109 | 0 |
1940 2-6 Jan | F + UF + L | 4, 7, 11, 14, 15, 17 | 7 | 3 | 35 | 1 043 |
1909 20-28 Dec | F + FF + UF + L | 1, 3, 4, 5, 6, 9, 10, 11, 13, 14, 15, 16, 17, 18 | 37 | 4 | 679 | 478 |
1904 9-12 Feb | F + L | 1, 3, 13, 17 | 27 | 1 | 1 | 3 |
1865 25-26 Nov | F + FF | 11 | 21 | 0 | 0 | 0 |
Event type: F - Flood; FF - Flash flood; UF - Urban flood; L - Landslide. Portuguese Districts: 1 - Aveiro; 2 - Beja; 3 - Braga; 4 - Bragança; 5 - Castelo Branco; 6 - Coimbra; 7 - Évora; 8 - Faro; 9 - Guarda; 10 - Leiria; 11 - Lisboa; 12 - Portalegre; 13 - Porto; 14 - Santarém; 15 - Setúbal; 16 - Viana do Castelo; 17 - Vila Real; 18 - Viseu. Source: Zêzere et al., 2020
Madeira has also experienced devastating flash floods. Quintal (1999) documented 30 flash floods that led to fatalities or severe damage on the island from the early 19th century to the late 20th century. Especially noteworthy is the event of 9 October 1803, which resulted in the loss of 800 to 1000 lives in Funchal. In the 21st century, the island has witnessed catastrophic events, notably the incident on 20 February 2010, which had particularly adverse effects on Funchal and Ribeira Brava (Fragoso et al., 2012.)
The areas prone to flooding in mainland Portugal were surveyed during the early stages of the 1st National Water Plan (INAG, 2001), which comprised critical sections and key points subjected to flooding. In a more recent study, Santos and Reis (2018) assessed flood susceptibility for rivers throughout mainland Portugal. This assessment employed a multicriteria analysis approach, integrating three key conditioning factors: average slope, accumulated flow, and average relative permeability. The findings obtained were subsequently validated against historical records of flood-related losses as summarized in the DISASTER database.
A comprehensive approach to assess flood risk and flood risk drivers for the Portuguese municipalities was provided by Santos et al. (2020). A flood risk index was computed by combining the hazard with exposure and the social vulnerability. Furthermore, the flood hazard was calculated by considering stream flood susceptibility, flood-prone regions, weather types, and an extreme rainfall index (Santos et al., 2020). Municipalities situated in the northwestern region of the country and along the valleys of major rivers (e.g. Douro and Tagus) exhibit a notable convergence of heightened exposure and hazard levels. Conversely, social vulnerability exhibits a scattered distribution across the country.
Sinkholes
There are hundreds of sinkholes in Portugal formed mainly over limestone of Jurassic age. These sinkholes are located along two geomorphological regions: the western Mesocenozoic borderland (including Condeixa-Sicó-Alvaiázere Massif, Estremenho Limestone Massif, Montejunto Massif, and Arrábida Massif) and the southern Mesocenozoic borderland (specifically in the Algarve barrocal area). Most of these sinkholes are blocked or obstructed, and they have been examined primarily through the lens of geomorphological heritage (e.g. Brilha & Pereira, 2020; Rodrigues, 2020), rather than being comprehensively assessed as potential geohazards. The hazard mapping of karst phenomena along the Algarve coast, as presented by Forth et al. (1999), stands as an exception. In their study, these authors mapped evidence of sinkhole collapse, subsidence, and gully erosion occurring along the Algarve's coastal region, spanning from Salema to Burgau, primarily affecting calcarenites of Miocene age. The authors employed a heuristic approach, assigning weights to nine predisposing factors, to generate a comprehensive hazard map.
Soil erosion
The soil erosion in Portugal has been addressed by assessing the impacts of land use and cover type on runoff erosion (Nunes et al., 2011). In a recent paper, Roxo et al. (2023) evaluated the impacts of historical and contemporary changes in land use and land cover on soil erosion within the Serra de Mértola region. This study exploited a comprehensive dataset collected over 58 years from erosion plots at the Vale Formoso Erosion Centre, complemented by field geomorphological observations, the interpretation of aerial photographs, and Landsat images. The primary focus of Roxo and co-authors was to analyse the persistent consequences of land use and land cover changes on vegetation response and soil erosion. Specifically, they examined three key transitions: the conversion of land from agricultural or grazing use to abandonment, the reverse transition from abandonment to agricultural or grazing use, and transitions between different types of agricultural and grazing land. This study also introduced a conceptual model that elucidates how sediment yield varies over time, both on hillslopes and valley floors, within the context of these land use / land cover changes (Roxo et al., 2023).
A special emphasis has been directed towards the investigation of gully erosion phenomena, in the context of soil erosion research in Portugal. Thresholds for gully initiation and sedimentation were proposed by Vandekerckhove et al. (2000) for the Mediterranean Europe, including data obtained in two Portuguese study areas located in Alentejo and Trás-os-Montes. More recently, Martins et al. (2022) identified the soil penetration resistance, slope gradient, slope shape, and vegetation cover as major local factors controlling the spatial variation of gully erosion.
The susceptibility to gully erosion has been assessed at the basin scale using statistic methods and physically-based methods. Bergonse and Reis (2016) applied a regression-based model using the reconstructed pre-erosion topography to assess controlling factors of large gully systems. Fernandes et al. (2017) used the SIMWE model to assess susceptibility to bank gully erosion in Alto Douro Wine Region agricultural terraces.
Lourenço et al. (2012) pointed out the occurrence of accelerate soil erosion after wildfires in Portugal, describing two erosional events occurred in the summer 2006 in areas that were burnt in 2005. Folharini et al. (2023) evaluated soil loss on watersheds in the North and Central Portugal using machine learning models and identified the recurrence of fires as a major factor controlling soil erosion. In a recent paper, Parente et al. (2022) used the semi‑empirical Morgan-Morgan-Finney erosion model to evaluate the potential soil erosion following wildfires, considering different burn severity and climate scenarios. These authors proposed a post-fire soil erosion hazard map for the forest and shrubland areas, at the national scale. The predictive model was validated using independent field datasets. The highest post-fire soil erosion hazard value is estimated in north-central Portugal, in areas covered by eucalypt, pine, and shrubland (Parente et al., 2022).
Final remarks
Following pioneering and innovative efforts during the 1980s, scholarly attention towards geohazards in Portugal has progressively intensified throughout the last decade of the 20th century, gaining significant momentum throughout the 21st century. Several analytical methodologies have been employed to explore hazardous phenomena, encompassing physically-based models, data-driven models, and heuristic techniques.
The corpus of published works dedicated to geohazards in Portugal has contributed to a better knowledge of the underlying physical processes of these hazards and their conditioning and triggering factors. In several cases, comprehensive assessments have been undertaken, encompassing susceptibility evaluation, as well as the determination of both the probability of occurrence and the magnitude of hazardous events. This approach has yielded comprehensive hazard assessments, which have been applied to several geohazards.
In certain scholarly investigations, the exploration of geohazards extended beyond the hazard assessment and ventures into the realm of risk analysis. In these instances, assessments encompass the examination of exposure levels, the valuation of assets at risk, and the assessment of vulnerability of exposed elements, encompassing both physical and social dimensions of vulnerability.
In terms of the geographical distribution of geohazards in Portugal, the western and southern coastal regions exhibit a heightened susceptibility to a broader spectrum of geohazard threats. Specifically, the Lisbon region, together with the Lower Tagus Valley and the Algarve, are the zones most prone to geohazards, encompassing hazardous processes such as earthquakes, tsunamis, coastal erosion, floods, flash floods, and landslides. Significantly, these high-hazard zones largely overlap with the country's most densely populated areas and encompass most of its economic activities and critical infrastructure. Civil protection stakeholders have acknowledged this high exposure to geohazards, particularly concerning earthquake and tsunami. Detailed studies have been conducted for both the Lisbon Metropolitan Area and the Algarve addressing the seismic and tsunami risks (Autoridade Nacional de Proteção Civil [ANPC], 2009, 2010).
In the rest of mainland Portugal, the inland North and Centre regions are more susceptible to landslides and soil erosion, in contrast to the inland South (Alentejo), which represents the safest zone concerning geohazards. However, it is important to note that the Alentejo region is highly susceptible to soil erosion, which contributes to the overarching threat of desertification.
In the Atlantic islands, the Madeira exhibits a marked susceptibility to landslides, flash floods, and coastal erosion, while the Azores archipelago presents a comprehensive array of geohazards, including active volcanoes, earthquakes, tsunamis, landslides, flash floods, and coastal erosion.