INTRODUCTION
Biostimulants are applied to plants or to the rhizosphere in order to enhance the natural process, improving the absorption of nutrients and the efficiency, quality and tolerance of crops to abiotic stresses (du Jardin, 2015). In soil they have shown a positive effect over the soil biological fraction, having a direct implication over the soil fertility (Caballero et al., 2020). Changes produced by biostimulants can be monitorized studing the nutrient-recycling enzymes expressed by the soil microbiota, that act as indicators of the quality and state of fertility of soil, and at microbiological level, thanks to metabarcoding techniques using 16S rRNA sequencing (Parlapani et al., 2018).
Sewage sludge, the organic by-product resulted from the treatment of wastewater, suposes a serious environmental issue. Scientific community is constantly searching for new alternatives uses. Considering sluge as raw material for the formulation of biostimulants is gaining interest as long as it does not exceed limit values for organic pollutants, nor heavy metals and it is sanitized in order to eliminate its pathogenic microorganisms.
Biostimulants, obtained by enzymatic or fermentative technology from different substrates, and composed by low molecular weight peptides and free amino acids, microbial metabolites, such as phytohormone analogues, polysaccharides, humic substances, beneficial microorganisms, etc., have shown positive effects on soil biostimulation and bioremediation of soil contaminants (Tejada et al., 2013, 2014).
Recent results in our group revealed that exogenous aplication of subtilisine from Bacillus, a naturally-produced-in-soil enzyme, produced stimulation of soil enzymes and changes in microbial biodiversity, favouring specific PGPR species (Caballero et al., 2020). Thus, a Bacillus-fermented sludge, composed by such enzyme and by a broad spectrum of other enzymes, would lead to interesting changes.
Our purpose is to evaluate the soil biostimulating capacicity, both at biochemical and microbiological level, of a Bacillus-fermented sludge obtained by fermentative technology.
MATERIAL AND METHODS
Biostimulant obtention and experimental design
Biostimulant products were obtained through a physical-fermentative process as described by Rodriguez-Morgado et al. (2019) (Figure 1).
FS product contains a total dry matter of 63.1 ± 0.17 g L-1, being 14.6% soluble matter. Bacterial concentration (B. licheniformis) in FS is 2.01 x 108 ± 1.11 x 108 CFU g-1. IFS (insoluble fraction) is composed by the Bacillus biomass and by the hydrolyzed insoluble organic matter; SFS (soluble fraction) is composed by the enzymatic secretion of Bacillus and by highly bioavailable soluble hydrolyzed organic matter, mainly composed by peptides and free aminoacids.
Experimental design was stablished according to previous studies (Caballero et al., 2020). Thus, microcosms of 250 g of soil were preincubated at 30-40 % at water holding capacity for 7 days. After this phase each product were added (0.1 and 0.5 % w/w) to soil under the following experimental conditions:
C: Control
SFS: Addition of FS
SIFS: Addition of IFS
SSFS: Addition of SFS
Analytical techniques
Proteomic study: Sample preparation and LC-MS analysis were carried out following the procedure described by Parrado et al. (2014).
Metabarcoding analysis: Soil DNA extraction, Illumina MiSeq sequencing and the analysis of the microbial community composition was performed as described in a previous work (Macias-Benitez et al., 2020).
Determination of soil enzymatic activities
Dehydrogenase activity was measured as described by García et al.; phosphatase activity was determined as described by Tabatabai and Bremner.
Statistical analysis
Soil enzymatic activities were compared using a one-way analysis of variance (ANOVA), followed by a Tukey test. Level of significance was established at p <0.05.
RESULTS AND DISCUSSION
Enzimatic characterization of the biostimulant
LC-MS analysis reported that the enzimatic component present in the soluble fraction of the biostimulant is comprised by proteins with hydrolitic and transport functions (Information not shown). Secreted hydrolases were 50% peptidases and amidases, related to N metabolism, and 33.3% glucanases, related to carbohydrate metabolism.
Evaluation of the biostimulant capacity in soil
Changes at biochemical level
It was observed that the soluble fracion of fermented sludge (SFS), and to a lesser extent the complete product (FS), produced the greatest stimulation on the soil biological activity (dehydrogenase activity SFS 0.5 % w/w: 2,89 ± 0,10 mmol INTF g-1 h-1; FS 0.5 % w/w: 1,66 ± 0,23 mmol INTF g-1 h-1, Figure 2).
In all cases, maximum peaks of stimulation were reached on day 5. The main reason to such stimulation is the molecular size profile of the soluble organic component of fermented sludge, constituted 65.95 ± 0.09% by organic molecules of molecular size under 1 KDa, mainly peptides, free amino acids and other highly bioavailable organic molecules, easily assimilated by the soil microbiota (Estrada et al., 2013).
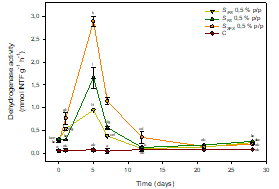
Figure 2 Dehydrogenase activity in controls and soils treated with biostimulants. Points (mean ± SD) with the same letter(s) are not significantly different from each other (p>0.05).
Also notable was the stimulation produced by SFS on phosphatase activity coinciding with the peak of dehydrogenase activity (SFS 0.5 % w/w: 0.46 ± 0,02 mmol PNF g-1 h-1, Figure 3), explained by the depletion of phosphorus as a consequence of the stimulation of the soil microbiota, by synthesing microbial phosphatases to make it more bioavailable.
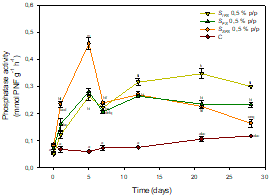
Figure 3 Phosphatase activity in controls and soils treated with the biostimulants. Points (mean ± SD) with the same letter(s) are not significantly different from each other (p>0.05).
Changes at microbiological level
Biostimulants produced changes within the soil microbial structure compared to the control, causing the stimulation of some bacterial genera bibliographically classified as beneficial microbes.
Showing a low presence in control samples, the relative abundance (RA) of Oxalobacteriaceae family, which encompasses several endophytic bacteria classified as plant growth promoters (PGPB) (Lambrecht et al., 2000), was induced at 5 days by the three treatments (increase in RA of 10.1%, 7.9% and 8.9% for TFS, SFS and IFS respectively). Within this family, the endophytic genus Herbaspirillum, includes nitrogen-fixing species, producers of phytohormones, such as gibberellin and auxin and siderophores, and have the ability to solubilize inorganic phosphorus among other PGPB capabilities (Rosconi et al., 2013).
Comamonadaceae family also was clearly induced (increase in RA of more than 50 %) by TFS and SFS treatments.
Maybe the most drastic change was found by SFS in the Moraxellaceae family coming to suppose 18.3% of total RA at five days. This change is entirely due to the increase of genus Acinetobacter that includes certain PGPB involved on the production of hormones, solubilization of phosphate, and production of siderophores. In addition, other strains of Acinetobacter exhibit indirect PGPR activity via the growth suppression of phytopathogenic fungi, and potential biocontrol properties against pathogenic bacteria (Xue et al., 2009).
Regarding to Bacillaceae family, it is represented by the genus Bacillus known to exert PGPR activity, protecting plants from phytopathogen and simultaneously increase the yield in different crops (Elanchezhiyan et al., 2018). As expected, it shows the highest RA at the beginning in TFS and IFS treated soils, decreasing along the time, while oppositely, in SFS treatment, it increases reaching similar values to TFS and IFS soils at five days (10.84% of total relative abundance).