Serviços Personalizados
Journal
Artigo
Indicadores
-
Citado por SciELO
-
Acessos
Links relacionados
-
Similares em SciELO
Compartilhar
Portuguese Journal of Nephrology & Hypertension
versão impressa ISSN 0872-0169
Port J Nephrol Hypert vol.31 no.3 Lisboa set. 2017
REVIEW ARTICLE
Glucosylceramides and kidney disease
Alberto Ortiz1,2, Maria Dolores Sanchez‑Nino1,2
1 IIS‑Fundacion Jimenez Diaz, School of Medicine, UAM, Madrid, Spain.
2 REDINREN, Madrid, Spain
ABSTRACT
Glucosylceramides are part of the wider family of glycosylceramides. Glucosylceramide synthase catalyzes the incorporation of a single glucose residue into ceramide to yield glucosylceramide. Glucosylceramide is known as a precursor for globotriaosylceramide (Gb3). In the cell membrane, Gb3 acts a receptor for verotoxin and plays a key role in allowing verotoxin entry into endothelial cells. Verotoxin causes endothelial cell injury leading to microangiopathy in hemolytic uremic syndrome. Additionally, Gb3 accumulates mainly in lysosomes in Fabry disease, caused by an X‑linked deficiency in alfa‑galactosidase A. Accumulated Gb3 is metabolized to lyso‑Gb3, a circulating water soluble molecule that elicits a stress response in podocytes similar to the response elicited by high glucose levels. The activation of Notch 1, CD74 expression and autocrine secretion of TGF‑β1 induced by lyso‑Gb3 and high glucose levels may underlie the characteristic fibrosis observed in both diabetic nephropathy and Fabry nephropathy. While current therapy for Fabry disease consists mainly of enzyme replacement therapy, glucosylceramide synthase inhibitors, such as lucerastat and venglustat, are undergoing clinical trials as substrate reduction therapy, aiming at decreasing Gb3 synthesis. Additionally, eliglustat is already in clinical use to treat Gaucher disease. Preclinical evidence has shown an excess accumulation of glycosylceramides in acute kidney injury and polycystic kidney disease. Furthermore, glucosylceramide synthase inhibitors are protective in polycystic kidney disease, suggesting a wider role of glucosylceramide or derivatives in kidney injury. However, inhibition of glucosylceramide synthase increases the severity of preclinical acute kidney injury, probably through increase ceramide accumulation, although direct toxicity of a specific drug could not be ruled out. This adds a note of caution for clinical studies, at least for some glucosylceramide synthase inhibitors.
Key words: Fabry Disease, Lyso‑Gb3, Diabetic Kidney Disease, Polycystic Kidney Disease, Acute Kidney Injury, Glucosylceramide, Glucosylceramide Synthase, Venglustat
WHAT ARE GLUCOSYLCERAMIDES?
Sphingolipids such as ceramide are converted by specific enzymes into glucosylceramides, which are glycosphingolipids with a single sugar residue. This sugar residue can be a glucose or a galactose, yielding glucosylceramide or galactosylceramide, respectively1.
Glucosylceramides are also called glucocerebrosides and are part of the wider family of glycosylceramides or cerebrosides. Glucosylceramide synthase catalyzes the incorporation of a single glucose residue into ceramide to yield glucosylceramide (Figure 1). Different glucosylceramides are characterized by different fatty acids in the fatty acid position.
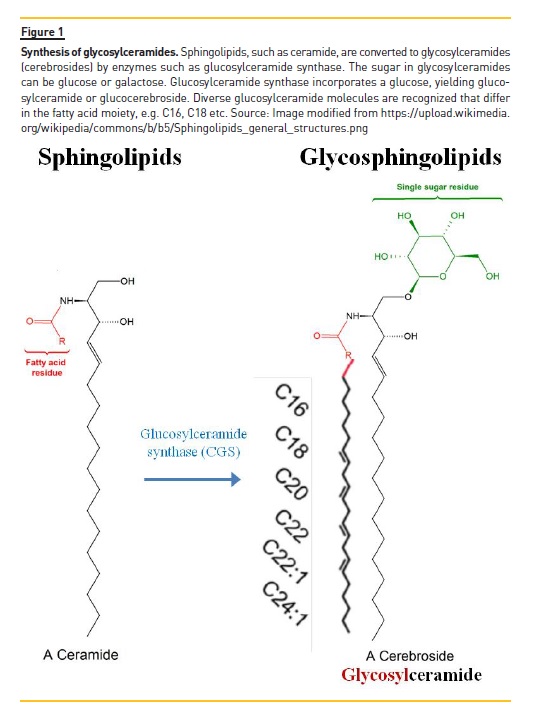
GLUCOSYLCERAMIDES AND KIDNEY DISEASE
Glucosylceramides are produced by the kidney in a sex‑dependent manner2. The kidneys of male mice express higher amounts of both galactosylceramides and glucosylceramides than the kidneys of female mice.
The kidney contents of both molecules increase suddenly in male mice from 20 to 30 days of age and remain increased in adulthood. Moreover, kidney glycosylceramide levels are increased in diverse forms of kidney disease. For example, in kidneys from MRL/lpr mice with lupus nephritis diverse glycosylceramide species are increased3. The assay used in this manuscript did not differentiate between glucosylceramides and galactosylceramides and, thus, the term glycosylceramides, encompassing both, is used. For example, increased levels of glycosylceramide 18:1/22:0; 18:1/24:0 and 18:1/24:1 were observed. Glycosylceramides are also increased in experimental animal models of acute kidney injury4. Thus, in cisplatin‑induced acute kidney injury, increased kidney levels of C16, C24:1 and C24 were observed. By contrast, levels of C18 and C20 glycosylceramides were not significantly different from control kidneys. In another toxic model of acute kidney injury, in this case resulting from an endogenous nephrotoxin (myoglobin), induced by intramuscular glycerol administration, causing rhabdomyolysis, increased levels of C16, C20, C24, and C24:1. By contrast, levels of C18 and C22:1 glucosylceramides were not significantly different from control kidneys. In ischemia/reperfusion‑induced acute kidney injury, the increased glycosylceramide species included C16, C24, and C24:1. By contrast, C18, C20 and C22:1 were not significantly different from control. In summary, the kidney contents of diverse glycosylceramide species are increased in preclinical acute kidney injury. Some glycosylceramide species were found increased in the three models tested (e.g. C16, C24 and C24:1). By contrast, other species, such as C20, were only found increased in some models. This suggests that within the common theme of increased kidney glycosylceramide levels during acute kidney injury, differences that may be related to etiology may be found. Potentially more interesting is the finding of increased glucosylceramide levels in polycystic kidneys, both from humans and from experimental animal models5.
Indeed, in human polycystic kidney disease, male sex is a risk factor for progression of the increase in total kidney volume, secondary to increased kidney cyst size, as well as for the progressive loss of renal function6.
We should remember the higher kidney glucosylceramide levels in males as compared to females, at least in mice2.
GLYCOSPHINGOLIPIDS AND FABRY DISEASE
Glucosylceramides are the precursors of glycosphingolipids that accumulate in Fabry disease.
Fabry disease is a hereditary systemic disease caused by deficiency of the GLA gene encoding lysosomal alpha‑galactosidase A7,8. The GLA gene is encoded by the X chromosome. Thus, Fabry disease is X‑linked.
Males have a severe disease, while the severity of Fabry disease in females is widely variable, depending on the random X chromosome inactivation within each cell. The clinical manifestations of Fabry disease start in childhood, and are characterized by neuropathic pain (traditionally termed acroparesthesia), hypohidrosis, abdominal pain and diarrhea, and a characteristic skin lesion, the angiokeratoma. These symptoms decrease the quality of life. However later manifestations may decrease survival. In adulthood,
Fabry patients develop a progressive proteinuric kidney disease that leads to the need for renal replacement therapy at a mean age of 40 years, both in males and in the few females that progress to this stage9-12. Additionally, in the third decade of life, left ventricular hypertrophy develops and progresses to a hypertrophic cardiomyopathy characterized by a typical myocardial scarring pattern in contrast‑enhanced magnetic resonance imaging. Patients may develop arrhythmia and heart failure. Finally, central nervous system involvement includes the development of white matter lesions as well as ischemic events (transient ischemic attack or stroke).
Current therapy of Fabry disease consists of the replacement of the missing enzyme or, for certain mutations, a chaperone that increase the activity of the abnormal protein. Two forms of human recombinant alpha galactosidase A, agalsidase alfa and agalsidase beta, are available. However, agalsidase beta was not authorized by the United States Federal Drug Administration to be commercialized in the United States. The drug is administered intravenously every two weeks. Surprisingly, the dose of agalsidase beta is 5‑fold higher than the dose of agalsidase alfa.
The reason for this discrepancy is unclear. While agalsidase beta was tested in 3 different doses (0.3, 1.0 and 3.0 mg/Kg biweekly) in phase I clinical trials and, based on the results of these dose‑findings trials, the 1.0 mg/Kg dose was chosen for pivotal clinical trials; agalsidase alfa was tested in dose‑finding clinical trials at a maximal dose of 0.1 mg/Kg (reviewed in7,8). Thus, the agalsidase alfa dose tested in pivotal trials was twice the maximal dose tested in phase I trials. We should remember here that phase I trials are mainly safety trials. In our view, there is no objective reason why 0.2 mg/Kg and not a higher dose was tested in pivotal trials. From the efficacy point of view, the dose response is unclear at higher agalsidase alfa doses. Data on long‑term efficacy of agalsidase preparations is scarce. The largest registry data reported to date, encompassing over 1000 patients, represents the experience acquired with agalsidase beta 1.0 mg/Kg biweekly in adults (median age 40 years) with classical Fabry disease, that is mutations known to be associated with non‑classical Fabry disease were excluded from the analysis13.
This report observed an approximately 50% decrease in the incidence rate of severe renal, cardiac and stroke events or death after 6 months of agalsidase therapy. This decrease in the incidence rate of severe clinical events persisted for the 5 years of the study and was observed both in high risk (males, those over 40 years old and those with a prior event, that is a severe clinical event occurring before starting enzyme replacement therapy) as well as in lower risk patients. In natural history, over the 5 years of follow‑up, an increase in the incidence rate of severe clinical events would have been expected, as patients got older and aging is the main risk factor for the type of events analyzed in the study. These data are consistent with the observed 50% decrease in the risk of severe clinical events in a phase IV clinical trial comparing agalsidase beta 1.0 mg/Kg biweekly with placebo. This trial had severe clinical events as primary end‑point7,8.
An ongoing clinical trial is comparing face‑to‑face agalsidase alfa 0.2 mg/Kg biweekly versus agalsidase beta 1.0 mg/Kg biweekly, with a primary end‑point of severe clinical events. The interim results at 8 years reported in 2016 were grossly underpowered (approximately six‑fold lower number of patients enrolled than expected)7,8. This precludes meaningful assessment of statistical significance.
However, the incidence of severe clinical events per patient was roughly double in patients randomized to agalsidase alfa than in those randomized to agalsidasa beta, despite the higher severity of Fabry disease at baseline in the agalsidase beta patients14.
The Cochrane collaboration assessed clinical trials of agalsidase in Fabry disease15. However, the analysis was marred by a paucity of clinical trials. A more recent analysis of cohort studies reported that agalsidase beta is associated to a significantly lower incidence of renal (pooled proportions for renal complications, agalsidase alfa 15.3% [95% CI 4.8, 30.3%]; agalsidase beta 6% [95% CI 4, 7%]; and untreated patients 21.4% [95% CI 15, 28%]), cardiovascular (agalsidase alfa 28% [95% CI 7, 55%]; agalsidase beta 7% [95% CI 5, 8%]; and untreated patients 26.2% [95% CI 14.9, 39%) and cerebrovascular events (agalsidase alfa 11.1% [95% CI 5.8, 17.9%]; agalsidase beta 3.5% [95% CI 2.4, 4.6%]; and untreated patients 18.3% [95% CI 12.9, 24.5) than no enzyme replacement therapy, and to a significantly lower incidence of cerebrovascular events than agalsidase alfa (agalsidase alfa 11.1% [95% CI 5.8, 17.9%]; agalsidase beta 3.5% [95% CI 2.4, 4.6%])16. Despite these favorable results with agalsidase beta, there is still margin for improvement. In part, this derives from the fact that Fabry disease is a rare disease; physicians do not immediately recognize its signs and symptoms, and diagnosis is frequently delayed for decades. Consequently, the median worldwide age at start of therapy (40 years) denotes that many patients start specific therapy for Fabry disease at an age when significant damage has already occurred in target organs.
The experience accumulated in diabetic nephropathy suggests that when end‑organ damage is already present, it is not sufficient to correct the metabolic defect, but additional add‑on therapies with tissue protective actions based on a clear understanding of the pathophysiology of the disease are required. Currently, anti‑proteinuric therapy with renin‑angiotensin‑system blockers is recommended for Fabry nephropathy, based mainly on the positive clinical experience obtained in diabetic nephropathy.
G LUCOSYLCERAMIDES AS PRECURSORS OF METABOLITES CONTRIBUTING TO THE PATHOGENESIS OF FABRY NEPHROPATHY
In Fabry disease, the low or inexistent alpha galactosidase A activity leads to the accumulation of globotriaosylceramide (Gb3, galactosyl‑galactosyl‑glucosylceramide) in lysosomes, since Gb3 is degraded by alpha galactosidase A (Figure 2). Gb3 is known to nephrologists as the receptor for shigatoxin. Inflammation is known to upregulate the enzyme Gb3 synthase.
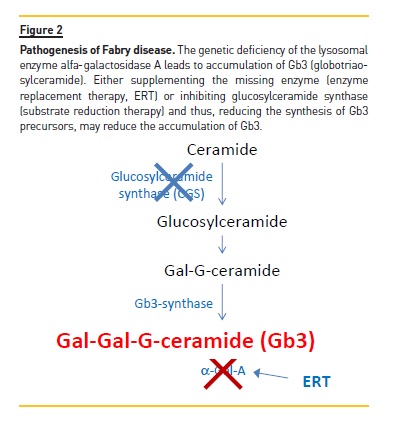
During hemolytic uremic syndrome induced by shigatoxin‑expressing E. coli, inflammation secondary to infection upregulates Gb3 synthase and, thus, Gb3 levels which are expressed in the cell surface of endothelial cells17. Shigatoxin uses Gb3 in the cell surface as a receptor to enter endothelial cells where it inhibits protein synthesis, leading to endothelial dysfunction and microangiopathy. In Fabry disease, Gb3 accumulation occurs mainly within lysosomes, and thus the pathogenesis differs from hemolytic uremic syndrome.
Podocytes are the kidney cell types that accumulate the most Gb318. This is the result of the long life of podocytes, terminally differentiated cells that do not divide and are not usually replaced by younger cells. The huge amount of Gb3 accumulation in podocytes is consistent with the proteinuric nature of Fabry nephropathy. Pathological albuminuria observed in childhood is the earliest manifestation of Fabry nephropathy. Indeed, in children, podocyte Gb3 deposits correlate with proteinuria and foot process effacement was observed in a segmental manner in all glomeruli.
It is thus of interest to clear podocyte glycolipids deposits. However, given the huge glycolipid deposits in podocytes and their location outside of capillaries, where a parenterally administered recombinant protein does not access as well as to endothelial cells, a higher cumulative agalsidase dose is needed to clear podocytes19.
Thus, after 5 years of agalsidase therapy, podocytes were only cleared in patients receiving the higher agalsidase cumulative doses. By contrast, endothelial cells were cleared in all patients. This is a key observation, since pivotal clinical trials of agalsidase used endothelial deposits as primary or secondary endpoint and results may not apply to podocytes.
LYSO‑GB3: A GB3 DERIVATIVE WITH A PATHOGENIC ROLE IN PODOCYTE INJURY DURING FABRY DISEASE
The pathogenesis of cell injury in Fabry nephropathy was poorly characterized for many years. However, the description in 2008 of lyso‑Gb3 was a great advance20. Lyso‑Gb3 results from the loss of one fatty acid from Gb3. This reaction takes place in the lysosome and generates a more water soluble molecule with biological activity (Figure 3). Initially, lyso‑Gb3 was reported to be present in Fabry patients but not in healthy controls. More recent analysis with more sensitive methods disclosed that in Fabry disease plasma lyso‑Gb3 was increased 100‑ to 200‑fold
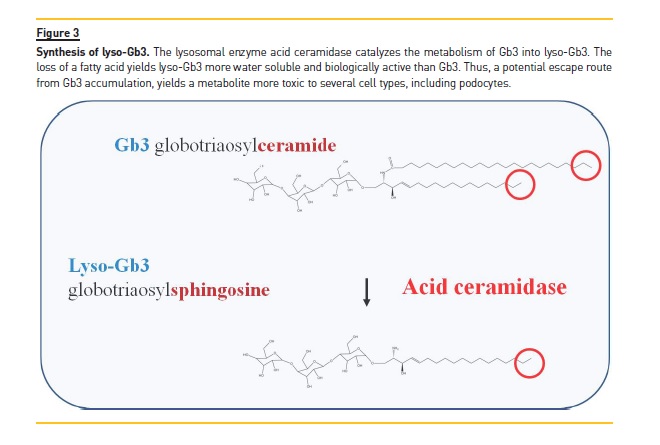
Over control values, as compared to a 2‑fold increase for Gb3. In the original description lyso‑Gb3, but not Gb3, was found to increase the proliferation of vascular smooth muscle cells. More recent data have identified lyso‑Gb3 as a key pathogenic factor that causes podocyte stress and injury21,22. Lyso‑Gb3, in a time‑ and dose‑dependent manner, and at concentrations found in Fabry patients, causes a stress response in cultured normal (non‑Fabry) podocytes very similar to the stress response elicited by high glucose levels. (Figure 4) Thus, lyso‑Gb3 leads to the autocrine secretion of TGF‑β1 that, in turn, promotes the expression of extracellular matrix proteins such as fibronectin and type IV collagen21. This profibrotic activity can be prevented by activators of vitamin D receptors, suggesting that vitamin D deficiency should be avoided in these patients21,23. Additionally, lyso‑Gb3 increases the mRNA expression and activation of Notch122. Notch1 activation in podocytes is known to promote glomerulosclerosis in vivo (Reviewed in24. Moreover, in diabetic nephropathy and in focal segmental glomerulosclerosis Notch1 is activated in podocytes (Reviewed in24. As a consequence of lyso‑Gb3 activation of Notch1, podocytes increased the expression of HES1, a canonical Notch1 target that is known to promote cell dedifferentiation22. Additionally, activation of Notch1 leads to activation of the proinflammatory transcription factor NFkB and the expression of inflammatory chemokines such as MCP‑1 and RANTES. These effects were observed in both cultured human podocytes and in vivo in wild type mice in whom lyso‑Gb3 promoted kidney infiltration by macrophages. The description of the pathogenic role of lyso‑Gb3 and the molecular mechanisms involve opens new avenues for the treatment of Fabry nephropathy. In this regard, g‑secretase inhibitors prevent Notch1 activation and the inflammatory response in podocytes exposed to lyso‑Gb3.
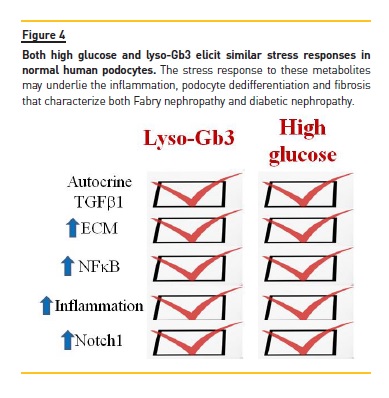
Evidence of Notch1 activation was also observed in human renal biopsies of Fabry nephropathy, including the expression of active Notch1 in podocytes as well as the expression of canonical Notch1 transcriptional target HES1 also in podocytes. Interestingly, higher doses of agalsidase better clear lyso‑Gb3 from the circulation of Fabry patients25.
The stress response elicited by lyso‑Gb3 in podocytes is similar to the response elicited by high glucose levels26-28 (Figure 4). The activation of Notch 1 and the autocrine secretion of TGF‑β1 may underlie the characteristic fibrosis observed in both diabetic nephropathy and Fabry nephropathy29. High glucose and lyso‑Gb3 also increase the podocyte expression of CD74, a mediator of glomerular injury that behaves as a receptor for the cytokines MIF and MIF‑221,30-32.
GLUCOSYLCERAMIDE SYNTHASE INHIBITORS IN FABRY DISEASE
The large size of agalsidase impairs its ability to reach cells outside the circulation, such as podocytes. Small molecules may reach the podocyte more easily. A search for small molecules that may be used to treat Fabry nephropathy identified glucosylceramide synthase as a potential target. This is the concept underlying substrate reduction therapy. In Fabry disease, an impaired drainage (GLA mutation) leads to accumulation of Gb3. It was hypothesized that decreasing the synthesis of Gb3 may also contribute to decreasing Gb3 levels. Glucosylceramide synthase generates glucosylceramide which is then further glycosylated by the addition of one galactose to yield Gb2 which in turn is further glycosylated by the addition of second galactose residue by Gb3 synthase to yield Gb3 (Figure 2). Glucosylceramide synthase inhibitors, including venglustat, have been shown to decrease Gb3 accumulation in kidneys from Fabry mice33,34 and two of them, lucerastat and venglustat, are undergoing clinical trials in Fabry patients35.
G LUCOSYLCERAMIDE SYNTHASE INHIBITORS FOR POLYCYSTIC KIDNEY DISEASE
However, the clinical use of glucosylceramide synthase inhibitors is not limited to Fabry nephropathy.
As discussed above, glucosyl or galactosylceramides also accumulate during acute kidney injury and in polycystic kidney disease. Glucosylceramide inhibitors have been tested in preclinical models of polycystic kidney disease and of acute kidney injury. In murine polycystic kidney disease glucosylceramide inhibitors prevented the accumulation of glucosylceramides in the kidneys, and slowed the increase in kidney cyst size and of the progressive loss of renal function5.
G LUCOSYLCERAMIDE SYNTHASE INHIBITORS AND ACUTE KIDNEY INJURY
By contrast to the beneficial effect observed in polycystic kidney disease, glucosylceramide inhibitors increase the severity of cisplatin‑induced kidney injury, leading to more severe kidney inflammation and higher urea levels4.
It was hypothesized that the predominant effect was an increase in ceramide levels that offset the potential beneficial levels of decreasing glucosylceramide, since ceramide synthesis inhibitors decreased both ceramide and glucosylceramide levels and were beneficial. In any case, this report raises the concern of the potential nephrotoxicity of at least some glucosylceramide synthase inhibitors.
Direct toxicity of a specific drug could not be ruled out. These concerns are reduced by the fact that eliglustat, a glucosylceramide synthase inhibitors already in clinical use to treat Gaucher disease, has so far proved safe from the kidney point of view.
CONCLUSIONS
Based on preclinical animal models, glucosylceramides are thought to play a key role as precursors of metabolites deleterious to the kidney in the context of polycystic kidney disease and Fabry disease. The glucosylceramide synthase inhibitor eliglustat is already in clinical use to treat Gaucher disease and two more inhibitors, lucerastat and venglustat, are undergoing clinical trials in Fabry patients. This will open the door for testing these drugs in further causes of kidney disease. Based on preclinical data, polycystic kidney disease is a good candidate for further studies on the nephroprotective actions of glucosylceramide synthase inhibitors. Verotoxin‑induced hemolytic uremic syndrome may also benefit from glucosylceramide synthase inhibitors. A note of caution is derived from the deleterious effect of a glucosylceramide synthase inhibitor in diverse models of acute kidney injury.
References
1. Ueda N. Sphingolipids in genetic and acquired forms of chronic kidney diseases. Curr Med Chem 2017; 24: 12381275. [ Links ]
2. Costantino‑Ceccarini E, Morell P. Synthesis of galactosylceramide and glucosylceramide by mouse kidney preparations. J Biol Chem 1973; 248: 82408246. [ Links ]
3. Nowling TK, Mather AR, Thiyagarajan T et al. Renal glycosphingolipid metabolism is dysfunctional in lupus nephritis. J Am Soc Nephrol 2015; 26: 14021413. [ Links ]
4. Dupre T V, Doll MA, Shah PP et al. Inhibiting glucosylceramide synthase exacerbates cisplatin‑induced acute kidney injury. J Lipid Res 2017; 58: 14391452. [ Links ]
5. Natoli TA, Smith LA, Rogers KA et al. Inhibition of glucosylceramide accumulation results in effective blockade of polycystic kidney disease in mouse models. Nat Med 2010; 16: 788792. [ Links ]
6. Heyer CM, Sundsbak JL, Abebe KZ et al. Predicted mutation strength of nontruncating PKD1 mutations aids genotype‑phenotype correlations in autosomal dominant polycystic kidney disease. J Am Soc Nephrol 2016; 27: 28722884. [ Links ]
7. Ortiz A, Sanchez‑Nino MD. Diagnosis and treatment of Fabry disease. Med Clin (Barc) 2017; 148: 132138. [ Links ]
8. Ortiz A, Sanchez‑Nino MD. Reply. Med Clin (Barc) 2017; 149: 271272. [ Links ]
9. Ortiz A, Cianciaruso B, Cizmarik M et al. End‑stage renal disease in patients with Fabry disease: natural history data from the Fabry Registry. Nephrol Dial Transplant 2010; 25: 769775. [ Links ]
10. Ortiz A, Oliveira JP, Waldek S et al. Nephropathy in males and females with Fabry disease: cross‑sectional description of patients before treatment with enzyme replacement therapy. Nephrol Dial Transplant 2008; 23: 16001607. [ Links ]
11. Warnock DG, Ortiz A, Mauer M et al. Renal outcomes of agalsidase beta treatment for Fabry disease: role of proteinuria and timing of treatment initiation. Nephrol Dial Transplant 2012; 27: 10421049. [ Links ]
12. Wanner C, Oliveira JP, Ortiz A et al. Prognostic indicators of renal disease progression in adults with Fabry disease: natural history data from the Fabry Registry. Clin J Am Soc Nephrol 2010; 5: 22202228. [ Links ]
13. Ortiz A, Abiose A, Bichet DG et al. Time to treatment benefit for adult patients with Fabry disease receiving agalsidase β: data from the Fabry Registry. J Med Genet 2016; 53: 495502. [ Links ]
14. http://garrodsymposium.com/garrod2016/posters/#p104;.accessed July 18, 2016. [ Links ]
15. El Dib R, Gomaa H, Carvalho RP et al. Enzyme replacement therapy for Anderson‑Fabry disease. Cochrane Database Syst Rev 2016; 7: CD006663. [ Links ]
16. El Dib R, Gomaa H, Ortiz A, Politei J, Kapoor A, Barreto F. Enzyme replacement therapy for Anderson‑Fabry disease: A complementary overview of a Cochrane publication through a linear regression and a pooled analysis of proportions from cohort studies. PloS one 2017; 12: e0173358. [ Links ]
17. van Setten PA, van Hinsbergh VW, van der Velden TJ et al. Effects of TNF alpha on verocytotoxin cytotoxicity in purified human glomerular microvascular endothelial cells. Kidney Int 1997; 51: 12451256. [ Links ]
18. Tøndel C, Bostad L, Hirth A, Svarstad E. Renal biopsy findings in children and adolescents with Fabry disease and minimal albuminuria. Am J Kidney Dis 2008; 51: 767776. [ Links ]
19. Tøndel C, Bostad L, Larsen KK et al. Agalsidase benefits renal histology in young patients with Fabry disease. J Am Soc Nephrol 2013; 24: 137148. [ Links ]
20. Aerts JM, Groener JE, Kuiper S et al. Elevated globotriaosylsphingosine is a hallmark of Fabry disease. Proc Natl Acad Sci U S A 2008; 105: 28122817. [ Links ]
21. Sanchez‑Nino MD, Sanz AB, Carrasco S et al. Globotriaosylsphingosine actions on human glomerular podocytes: Implications for Fabry nephropathy. Nephrol Dial Transplant 2011; 26: 17971802. [ Links ]
22. Sanchez‑Nino MD, Carpio D, Sanz AB, Ruiz‑Ortega M, Mezzano S, Ortiz A. Lyso‑Gb3 activates Notch1 in human podocytes. Hum Mol Genet 2015; 24: 57205732. [ Links ]
23. Terryn W, Cochat P, Froissart R et al. Fabry nephropathy: indications for screening and guidance for diagnosis and treatment by the European Renal Best Practice. Nephrol Dial Transplant 2013; 28: 505517. [ Links ]
24. Sanchez‑Nino MD, Ortiz A. Notch3 and kidney injury: never two without three. J Pathol 2012; 228: 266273. [ Links ]
25. Goker‑Alpan O, Gambello MJ, Maegawa GHB et al. Reduction of plasma globotriaosylsphingosine levels after switching from agalsidase alfa to agalsidase beta as enzyme replacement therapy for fabry disease. JIMD reports 2016; 25: 95-106. [ Links ]
26. Perez‑Gomez MV, Sanchez‑Nino MD, Sanz AB et al. Targeting inflammation in diabetic kidney disease: early clinical trials. Expert Opin Investig Drugs 2016; 114. [ Links ]
27. Perez‑Gomez MV, Sanchez‑Nino MD, Sanz AB et al. Horizon 2020 in diabetic kidney disease: the clinical trial pipeline for add‑on therapies on top of renin angiotensin system blockade. J Clin Med 2015; 4: 13251347. [ Links ]
28. Sanchez‑Nino M‑D, Bozic M, Cordoba‑Lanus E et al. Beyond proteinuria: VDR activation reduces renal inflammation in experimental diabetic nephropathy. Am J Physiol Renal Physiol. 2012; 302: F647F657. [ Links ]
29. Weidemann F, Sanchez‑Nino MD, Politei J et al. Fibrosis: a key feature of Fabry disease with potential therapeutic implications. Orphanet J Rare Dis 2013; 8: 116. [ Links ]
30. Valino‑Rivas L, Baeza‑Bermejillo C, Gonzalez‑Lafuente L, Sanz AB, Ortiz A, Sanchez‑Nino MD. CD74 in kidney disease. Front Immunol 2015; 6: 483. [ Links ]
31. Sanchez‑Nino MD, Sanz AB, Ruiz‑Andres O et al. MIF, CD74 and other partners in kidney disease: tales of a promiscuous couple. Cytokine Growth Factor Rev 2013; 24: 2340. [ Links ]
32. Sanchez‑Nino MD, Sanz AB, Ihalmo P et al. The MIF receptor CD74 in diabetic podocyte injury. J Am Soc Nephrol 2009; 20: 353362. [ Links ]
33. Abe A, Gregory S, Lee L et al. Reduction of globotriaosylceramide in Fabry disease mice by substrate deprivation. J Clin Invest 2000; 105: 15631571. [ Links ]
34. Ashe KM, Budman E, Bangari DS et al. Efficacy of enzyme and substrate reduction therapy with a novel antagonist of glucosylceramide synthase for fabry disease. Mol Med 2015; 21: 389399. [ Links ]
35. Guérard N, Oder D, Nordbeck P et al. Lucerastat, an iminosugar for substrate reduction therapy: tolerability, pharmacodynamics, and pharmacokinetics in patients with fabry disease on enzyme replacement. Clin Pharmacol Ther 2017. [ Links ]
Alberto Ortiz
Division of Nephrology IIS‑Fundacion Jiménez Díaz
Av Reyes Católicos 2
28040 Madrid, Spain
Tel: +3491 5504940
e‑mail: aortiz@fjd.es
Funding: This work was supported by FIS PI16/02057, PI15/00298, CP14/00133, FEDER funds ISCIII‑RETIC REDinREN RD16/0009, Sociedad Española de Nefrología, Programa Intensificacion Actividad Investigadora (ISCIII/Agencia Lain‑Entralgo/CM) to AO, Miguel Servet MS14/00133 to MDSN.
Disclosure of potencial conflicts of interest:Alberto Ortiz is consultant for Genzyme, a Sanofi company, and has received speaker fees from Shire and Amicus. Maria Dolores Sanchez Niño has received speaker fees from Genzyme, a Sanofi company.
Received for publication: Sep 27, 2017
Accepted in revised form: Sep 30, 2017