Serviços Personalizados
Journal
Artigo
Indicadores
-
Citado por SciELO
-
Acessos
Links relacionados
-
Similares em SciELO
Compartilhar
Portuguese Journal of Nephrology & Hypertension
versão impressa ISSN 0872-0169
Port J Nephrol Hypert vol.32 no.1 Lisboa mar. 2018
REVIEW ARTICLE
Clinical implications of anti-HLA antibodies testing in kidney transplantation
Jorge Malheiro1, Sandra Tafulo2
1 Nephrology & Kidney Transplantation Department, Centro Hospitalar do Porto, Hospital de Santo António, Porto, Portugal
2 HLA Laboratory, Centro de Sangue e de Transplantação do Porto, Instituto do Sangue e Transplantação, Porto, Portugal
ABSTRACT
Alloantibodies against donor human leukocyte antigens (HLA), termed as donor‑specific antibodies (DSA), are one of the most important factors for both early and late kidney allograft dysfunction. In the past, these antibodies were mainly detected through cell‑based crossmatch tests. Recently, new techniques such as solid phase immunoassays (SPI) have revealed these antibodies in patient sera with a high degree of detail, previously unimaginable. They have allowed us to accurately determine recipients allosensitization status, improve pre‑transplant risk assessment with a potential donor and post‑transplant alloimmune monitoring. However, the high sensitivity of these new assays has also created areas of uncertainty about their clinical impact.
In the pre‑transplant setting, the presence of preformed DSA has been associated with an increased risk of antibody‑mediated rejection (AMR) and subsequent allograft loss. Nevertheless, several studies have shown that not all DSA are deleterious. Hence, understanding the clinical correlations of DSA characteristics, namely strength, HLA class, complement‑fixing ability or IgG subclasses, is paramount for an adequate stratification of the immunological risk at transplant. Furthermore, given that the number of allosensitized patients on waiting lists is increasing, the added information from these new SPI is essential to improve their chance of being transplanted with an admissible immunological risk.
After transplantation, the appearance of de novo DSA (dnDSA) has also been associated with a deleterious effect on kidney allograft survival. Moreover, it has been acknowledged that a majority of late allograft failures are caused by alloantibody‑driven injury. The current challenges, in this setting, are determining cost‑effective DSA screening protocols and understanding which patients could benefit from specific interventions. Furthermore, although therapeutic strategies to control antibody‑induced damage remain limited, the longitudinal surveillance of dnDSA emergence and the clinical correlations of their characteristics will play a crucial role in the improvement of late kidney allograft survival.
Keywords: allosensitization, cell‑based crossmatches, donor‑specific antibodies, kidney transplantation, solid‑phase immunoassays
INTRODUCTION
Despite improvements in patient selection and management, most transplants carry some immunological risk. Alloantibodies against human leukocyte antigens (HLA), chiefly donor‑specific antibodies (DSA), either present at the time of transplantation or arising de novo post‑transplant, are a risk factor for antibody mediated rejection (AMR) and potentially kidney allograft loss1. Hence, avoiding DSA at transplant is a desirable objective, although not always possible to attain, particularly in highly‑sensitized (HS) patients. Moreover, it has been demonstrated that patient survival is improved with HLA‑incompatible strategies in comparison to waiting on dialysis for a HLA‑compatible allograft2.
Our aims in this review are to provide a practical guide for using solid phase immunoassays (SPI) and crossmatch (XM) testing, either before or after kidney transplantation, and to contribute to a better understanding of their clinical implications.
TECHNICAL ASPECTS
Kidney transplantation (KT) requires a thorough immunological study between donor and recipient pair (Table 1).
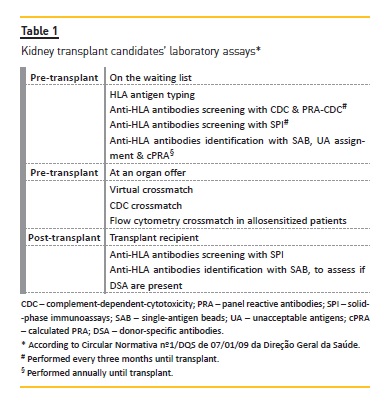
HLA Typing
HLA typing was first performed by lymphocytotoxic assays3 but since polymerase‑chain‑reaction (PCR) description in 19904, molecular methods have improved greatly and performing HLA typing at the antigen level for HLA‑A, ‑B and DRB1 loci is now mandatory for all donors and recipients pairs. Extended typing for HLA‑C, ‑DQ and/or DP should also be performed to assess complete degree of matching between the pair, especially if the recipient has alloantibodies against any of these loci.
The methods employed are sequence‑specific‑primer (SSP)5, real‑time PCR (qPCR), reverse sequence‑specific oligonucleotide (rSSO)6 and sequence‑based typing (SBT)7. The choice of the method depends on the resolution needed, how urgent the need for the results is and the number of samples to process.
Recently, a new technology of next generation sequencing (NGS) has been introduced in histocompatibility laboratories, allowing allelic level typing with high throughput8, an improvement still controversial9.
Although it would allow better HLA matching, with an indisputable role in reducing sensitization, improving allograft survival10, and clearly benefiting sensitized patients11, it would also prevent transplants offers for rare alleles due to the high polymorphism of HLA region12.
Understanding that transplanting all patients with a HLA full‑match allograft is an impossible goal, and that HLA mismatches are inevitable, it is our task to minimize their impact. In this context, the future for organ allocation systems can be HLA eplet matching by electing transplants with low HLA eplet mismatch (MM) load and avoiding highly immunogenic eplets13.
Besides matching, HLA typing is of extreme importance when performing the virtual crossmatch (vXM) at an organ offer (Table 1). A vXM is positive when a patient has HLA alloantibodies against a particular donor, i.e. DSA. A positive vXM usually is a contra‑indication for transplant but, depending on the locus involved, alloantibody strength, patient immunological history and sensitization status, it can be considered as a risk factor with appropriate immunosuppression strategies2,14.
Allosensitization status
About 2535% of patients on the waiting list for KT are pre‑sensitized15, mainly due to previous transplants, followed by pregnancies and transfusions16.
While on the waiting list, KT candidates must undergo alloantibody testing every three months and after each sensitizing event, with cellular and SPI (Table 1).
Cellular assays
Cellular assays are crossmatch tests between donor lymphocytes and recipient sera. To accomplish this, viable donor lymphocyte isolation is required, usually performed by density gradient centrifugation17. More recently, lymphocyte isolation with magnetic beads has been introduced with considerable improved results18.
Cell‑based crossmatches include complement‑dependent cytotoxicity (CDC) and flow cytometry crossmatch (FCXM).
Cytotoxic Crossmatch
CDC crossmatch (CDC‑XM) was first described by Terasaki and Patel in 196919. They showed that the presence of alloantibodies in recipient sera against antigens expressed on donor lymphocytes was a major risk factor for immediate allograft loss.
The assay consists of a first incubation (30 minutes) of recipient sera and donor cells in a micro‑well tray allowing, if present, DSA binding to donor cells. It can be performed from total lymphocyte population or after T‑and B‑lymphocytes sub‑populations separation.
Complement is then added following a second incubation (60 minutes), activating the complement classical pathway, resulting in lymphocyte lysis. The cell membrane loss of integrity is visualized with an inverted fluorescence microscope, after the addition of a vital dye. Percentage of cell lysis is recorded using the accepted International Histocompatibility Workshop (IHW) scoring system (0,1,2,4,6,8).
This methodology, called National Institute of Health (NIH) basic, standard or classic CDC‑XM, detects the presence of IgG1, IgG3 and IgM cytotoxic alloantibodies and is performed at an organ offer (Table 1), reducing hyperacute and early accelerated rejection episodes.
However, it has also been reported that some patients experienced early allograft loss despite a negative CDC‑XM.
To overcome this, several changes to the original technique were proposed to improve sensitivity, such as extended incubation times20, washing steps following first incubation removing unbound sera before adding the complement21, or amplifying complement activation and cell lysis with anti‑human globulin (AHG)22.
Nevertheless, these assays are also associated with false positive results due to clinically irrelevant non‑HLA antibodies and HLA IgM alloantibodies. The latter are frequent in patients with autoimmune disorders and can be overcome by treating recipients sera with dithiothreitol (DTT), reducing IgM disulfide bonds23. Also, autoreactive cytotoxic alloantibodies can generate a positive irrelevant CDC‑XM.
In these cases, to assist the interpretation of the allo‑XM, an auto‑XM is recommended24.
Flow Cytometry Crossmatch
Despite all uplifts in the classic CDC‑XM technique, it was only with FCXM that a true sensitivity boost was seen in cellular assays, allowing the detection of low level DSA25. For this reason, a positive FCXM with a negative CDC‑XM does not predict hyperacute rejection, but a lower allograft survival at 1‑year20.
This assay is performed at an organ offer for every sensitized patient and in most living donations (Table 1).
This methodology detects non‑complement fixing alloantibodies and was first described by Garovoy et al26. In 1989, Bray et al. described a dual‑color method27 and in 1996 a three‑color method was described by Robson et al.28. This assay consists of an indirect immunostaining where antibody‑antigen interaction is identified using an anti‑human immunoglobulin (F(ab)2 anti‑IgG) labeled with a fluorochrome. T‑ and B‑ lymphocyte subpopulations are identified using the monoclonal alloantibodies anti‑CD3 and anti‑CD19, labeled with fluorochromes with different emission wavelengths.
A positive reaction is calculated based on the median channel shift (MCS) between negative controls and patient sample using a cut‑off value established within each laboratory.
This technique has also been a subject of different improvements, with Lobo et al.29 describing that pronase treatment of the cells, prior to the FCXM assay, increased sensitivity and specificity30. It has also been shown that this treatment is effective in eliminating rituximab interference used for desensitization31.
Recently Liwski et al. investigated the impact of several assay parameters, such as incubation times and temperatures, cell number per reaction and serum: cell suspension volume ratio, developing of a rapid FCXM procedure: the Halifax and Halifaster protocols18. The Canadian group optimized protocols allow cost reduction with decreased assay time, without compromising sensitivity.
Solid‑phase immunoassays
SPI consist of solid‑phase platforms with purified HLA antigens covalently bound, such as the wells of a polystyrene microplates or microspheres. These tests are performed every three months before transplant, but also after transplantation ideally after the first, third, sixth month and then annually (Table 1).
ELISA
Enzyme‑linked immunosorbent assays (ELISA) has been described by several authors as an alternative method to cell‑based assays32. Soluble HLA (sHLA) antigens are affixed to the wells of microtiter plates.
HLA specific alloantibodies present in patient sera will bind to HLA antigen after being added to the well. This antibody‑antigen interaction is detected by the addition of an alkaline phosphatase‑conjugated with anti‑human immunoglobulin (IgG) antibody. A quantitative measure of the extent of reaction is obtained by spectrophotometric determination following the addition of the appropriate enzyme substrate for the color development.
Multi‑analyte profiling (xMAP®) technology
xMAP® technology is a multiplex assay that uses a panel of fluorescently dyed micron‑sized polystyrene microspheres, produced by the internal conjugation of variable amounts of two or three dyes, enabling the identification of 100 or 500 different beads, respectively.
HLA antigens are bound to these coded‑color beads and, after a first incubation with patients sera, any HLA alloantibodies present bind to the antigens on the beads. This reaction is detected, after a second incubation with R‑Phycoerythrin (PE)‑conjugated goat anti‑human IgG, with a Luminex® flow analyzer (LABScan 100 or LABScan3D) that simultaneously detects the fluorescent emission of PE and the dye signature from each bead. The light signal produced by bound is proportional to its concentration and is expressed as the mean fluorescence intensity (MFI).
The first xMAP® assay described used beads coated with HLA proteins extracted from individuals cells, allowing improved standardization in HLA alloantibodies detection. These beads can be composed by a pooled antigen panel screening assay 33 or by a single individual cell line phenotypic assays34.
Although a substantial improvement was brought to histocompatibility laboratories by these assays, it was still difficult to assign alloantibody specificity, especially for highly sensitized patients. To surpass this limitation, the same group published a modification of the assay that revolutionized allosensitization assessment35. This improved method consists of coated beads with a single class I or class II HLA recombinant antigen; the single antigen bead (SAB) assay. Since then, SAB has been the method of choice for unacceptable HLA antigen assignment in patients on the waiting list for deceased donor kidney transplantation, although the cut‑off value for positivity definition has been a challenge. Despite the 10001500 MFI value is usually taken into consideration, we must not forget that this is a semi‑quantitative assay and epitope and cross‑reactive groups (CREG) should be considered in the analysis36.
Classic SAB assay detects anti‑HLA alloantibodies of all IgG subclasses, but they are not equally detrimental.
To address this issue, a modification of the standard SAB IgG method to detect only complement‑binding HLA alloantibodies was developed37. In this test, human complement C1q is added in the first incubation with patient sera and HLA‑coated beads. The HLA alloantibodies bind to the target antigens, followed by attachment of C1q to alloantibodies that are complement‑binding.
An anti‑human C1q, conjugated with PE, is used as a reporter to indicate the presence of complement‑binding HLA alloantibodies when analyzed in a Luminex® instrument. Another assay to detect complement‑binding HLA alloantibodies was recently introduced into the market. This newest test uses an anti‑human C3d antibody, thus analyzing a different downstream step of the classic complement pathway than the former C1q assay38.
Although a certain degree of correlation between the amount of alloantibody and complement‑fixing activation should be expected, as at least six alloantibodies organized in hexamer are necessary to initiate the complement cascade39, the added value of this assay is debatable within the transplantation community.
Several authors have suggested that this assay might be redundant when compared to the MFI value in the classic IgG SAB assay, especially if confounding analytical interferences are corrected40.
Interpretative considerations
Although cellular assays are of undeniable importance, one should also understand their limitations. All cell‑based assays can give misleading results: false positivity, due to autoantibodies and non-HLA antibodies, and false negatives as a result of their lack of sensitivity for low titer alloantibodies.
With the newer SPI methods, laboratories intended to overcome these deficiencies. This technology proved to have several advantages over classic methods such as: i) elimination of the necessity for viable lymphocytes, allowing automation and becoming a high‑throughput laboratory method; ii) consistent and extended HLA panel, ensuring HLA antigen representativeness; iii) sensitivity, detecting low titer alloantibodies; iv) specificity, detecting only HLA IgG alloantibodies and v) clear distinction between HLA class I and class II alloantibodies (Table 2).
However, regardless of SPI assays unquestionable utility, in particular SAB assays that allow a greater accuracy in unacceptable HLA antigen assignment and the introduction of calculated panel reactive antibodies (cPRA), it is very important to understand that this methodology also has important limitations. SAB have limited HLA alleles, antigen density variations and cryptic epitopes exposure, resulting in false positivity due to denatured antigens42. This laboratory assay is also affected by immunomodulatory treatments such as intravenous immunoglobulin, resulting in increased background43, and ATG treatment, where usually an HLA‑A3 is identified because it is a polyclonal rabbit antibody raised against the Jurkat human T‑cell line (HLA‑A3,32; B7, 35)44. It also suffers from complement activating alloantibodies interferences that deposit C1 complex on the beads, or IgM alloantibodies, interfering with secondary antibody binding giving false negative results, called prozone effect. Several changes have been proposed in order to upgrade the test, such as dilutions, EDTA (ethylenediaminetetraacetic acid), DTT or heat treatment45.
All methods available should be considered in an integrated analysis (Table 3), along with history of sensitization, understanding that all techniques have different levels of sensitivity, detect different types of alloantibodies, and are subject to different interferences46.
As such, the decision to proceed with a KT should be the result of a multidisciplinary team effort between clinicians and histocompatibility laboratory to ensure the best immunological evaluation possible.
WAITLIST TESTING OF ANTI‑HLA ANTIBODIES
As described in Table 1, KT candidates in waitlist are tested quarterly, or after any sensitizing event, to assess allosensitization degree with panel reactive antibodies (PRA) value, and to assign unacceptable antigens (UA) for virtual crossmatch (vXM).
Defining unacceptable antigens
UA are assigned by SAB, using a reference cut‑off that is usually 1000 MFI, and by CDC. The definition of UA for all wait‑listed patients allows vXM determination, a preliminary in silico crossmatch that predicts positive crossmatches. The usefulness of this step is to avoid performing crossmatches that will be positive, although this is not an absolute contraindication per se.
Measuring transplantability
PRA is a measure of patients degree of sensitization while on the waiting list for transplantation, representing the percentage of the population to which the patient is sensitized47. Traditionally, it was performed by CDC using a panel of HLA typed donors, allowing the identification of UA, although with a high degree of inaccuracy since each cell expresses six alleles. A candidate for kidney transplantation with a PRA>85% is considered HS and usually is prioritized in allocation programs.
This classic PRA value depends greatly in the panel composition, that may not represent the antigen frequencies in the donor population, besides the fact that rare antigens are usually missed in the panel, and CDC intrinsic low sensitivity. For this reason, PRA does not provide an accurate measure of transplantability and, with the development of SAB assays, cPRA was introduced providing consistency48. Nowadays cPRA has replaced classic PRA in most kidney allocation systems worldwide, with great improvements in transplantation rates for sensitized patients49.
STRATIFYING THE RISK OF PREFORMED DSA
Several centers have a sizeable experience in performing kidney transplants in the presence of preformed DSA, in which the potential risk of AMR is considered acceptable14,50,51. They base their approach on the knowledge that not all DSA are equally pathogenic, with some of them being manageable through the use of increased immunosuppression or desensitization protocols. Hence, understanding DSA characteristics, namely strength (read as MFI), HLA class, complement‑fixing ability or IgG subclasses, is paramount for an adequate stratification of the immunological risk at transplant.
DSA strength
Although SAB assay was not approved as quantitative, it has been used clinically as a semiquantitative assay for the estimation of a given anti‑HLA alloantibody strength (measured as MFI). Several groups have shown that a higher DSA MFI is associated with AMR and allograft loss52-54.
Frequently, patients present at transplant with more than one DSA. So, determining if DSA strength measurement should consider the immunodominant DSA (highest MFI) or the MFI sum of all detected DSA (cumulative MFI) is an important, though still unanswered question.
Many centers opt to use one of them, since the comparison of their predicted behavior has not produced a clear result54,55. Recently, Zecher et al.53 showed, in non‑desensitized patients, that only DSA MFI above 10000 (immunodominant or cumulative) were associated with AMR occurrence and reduced allograft survival.
Our group has demonstrated that DSA strength had a good predictive performance for AMR occurrence, with a MFI >5000 in the immunodominant DSA having a sensitivity of 86% and a specificity of 73%55. Importantly, in our cohort, preformed DSA were associated with reduced kidney allograft survival only when AMR occurred. Similar results have been published by other groups52,54,56,57, although the MFI threshold to define DSA as clinically significant varied between 3000 and 10000 according to DSA number, HLA loci or the use of desensitization, limiting the reproducibility of these results. Alternatively, considering the pivotal role of HLA laboratory protocols, each center should work with their reference lab to define in‑house clinically significant DSA MFI thresholds. Furthermore, the full extent of the data given by SAB assays must be integrated with other variables, such as past sensitization events, the immunosuppression or desensitization used and the results of cell‑based crossmatches, namely flow cytometry58.
An alternative to DSA MFI, as a measure of alloantibody strength, is the determination of its titer, in which serial dilutions are performed until the alloantibody is no longer detected, corresponding that dilution to the alloantibody titer40. This approach is inherently closer not only to the alloantibody strength but also to its avidity, pertaining a closer representation of the in vivo antibody pathogenicity. Nevertheless, the clinical application of alloantibody titration in kidney transplantation remains largely undetermined and the high cost and labor involved prevents, at the moment, its broader use59.
DSA HLA class
Historically, it was considered that DSA against HLA class I was chiefly associated with early immunological events, while DSA against HLA class II were more important in the development of late (chronic) rejection60.
When detailed analyses of DSA by solid‑phase assays became available, published data demonstrated that both classes are equally pathogenic54. Moreover, DSA against HLA‑Cw,‑DQ or ‑DP, previously underappreciated, have shown to be responsible for the development of acute or chronic AMR and should be taken into account (61,62). Likewise, different groups have reported that the presence of DSA against both HLA classes poses a significantly higher risk of AMR and allograft failure52.
Complement‑binding DSA
The activation of complement is an important step in DSA‑driven allograft injury, starting with C1q binding and, downstream, C3 cleavage and production of C3d38. Modified SAB assays have been produced to detect DSA C1q‑ or C3d‑fixing ability63. Several studies have analyzed complement‑activating DSA ability correlation with its pathogenicity, with no clear‑cut results. Some demonstrated a strong association between C1q‑ or C3d‑binding DSA, AMR and allograft failure64,65, while others did not66,67. In the setting of preformed DSA, our group68 found that C1q and IgG MFI were both correlated with AMR. C1q status was better than IgG DSA strength of at least 15 000 MFI (OR=16.3 vs. 6.4, respectively) for predicting AMR. Furthermore, C1q+ DSA was a significant risk factor for AMR (OR = 16.80, P = 0.001) but high MFI DSA was not. Six‑year allograft survival was also significantly lower in high MFI C1q+ DSA in comparison with high MFI C1q−, or low MFI DSA (38, 83 and 80%, respectively; P = 0.001). Recently, similar results have been reported69.
However, an important drawback of these assays is their close relationship with DSA MFI and titer, limiting our ability in distinguishing the negative effects of complement‑activating DSA from its MFI70. Moreover, a large study has reported that C1q+ DSA was not associated with allograft failure when present only before transplant64.
DETAILING THE RISK OF DE NOVO DSA
Some of the observations referred above in relation to preformed DSA should be taken into account when analyzing the risk of de novo DSA (dnDSA), although the specificities of the latter merit a closer look of clinical data in this setting. One issue that remains under discussion is the timeframe of dnDSA detection post‑transplant. International guidelines published in 2013 (Table 4) indicate that, in the first‑year post‑transplant, all patients should undergo DSA screening at 3‑ and 12‑months, besides other clinically‑driven indications71.
A more intense screening schedule is proposed for patients with higher immunological risk (e.g., those desensitized). After 1‑year, they recommend the storage of serum every year, with DSA detection being performed when clinically indicated.
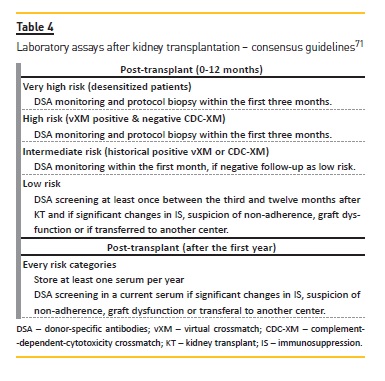
Incidence and clinical impact of de novo DSA
The negative impact of dnDSA on long‑term kidney allograft survival is widely recognized72. Moreover, when analyzing the causes of allograft failure, the Deterioration of Kidney Allograft Function (DeKAF) study showed that a majority of them was caused by rejection (64%), chiefly antibody‑mediated73.
Recently, in a case‑control study, we reported74 that DSA prevalence in patients with allograft failure (cases) was 56%, while only 16% in those remaining with a functioning allograft (controls).
Several recent longitudinal studies have detailed the role of dnDSA in allograft loss75,76. In previously non‑sensitized kidney allograft recipients, incidence of dnDSA at 1‑year was 11%, with an increase to around 20% at 5‑years76.
Wiebe et al.75 documented dnDSA in 15% of low‑risk patients at 4.6±3 years post‑transplant, with a 10‑year allograft survival of 57% in those with dnDSA and 96% in those without dnDSA.
Importantly, they also demonstrated that non‑adherence was the most important risk factor for de dnDSA emergence (OR=8.75, P<0.001). In a cohort of simultaneous pancreas‑kidney transplanted patients, we showed77 that dnDSA were detected in 15% at a median 3.1 years after transplant. dnDSA were significantly associated with kidney (in association with acute rejection) and pancreas allograft failure.
Clinical correlations of de novo DSA characteristics DSA strength
Data on the impact of alloantibody MFI on allograft outcomes in the setting of dnDSA is scarcer than in preformed DSA. Recently, a longitudinal study analyzing the rates of progression to allograft failure in patients with dnDSA, showed that DSA MFI was an independent predictor of failure (HR per 1000 MFI=1.02, P=0.029)78.
Heilman et al. observed that cumulative incidence of AMR or mixed rejection at 1 year was 30% in the group with dnDSA MFI >3000 but only 4% for the group with a MFI <300079. Others have shown that dnDSA MFI levels were associated with higher rate of acute AMR but did not have a significant impact on allograft survival80.
DSA HLA class
One of the striking observations, when studying dnDSA, is the clear predominance of antibodies against HLA class II. Wiebe et al.75 reported, in 47 patients dnDSA, that DSA anti‑HLA class I only were present in 3 patients, while 32 had anti‑HLA class II only and the remaining 12 had DSA against both HLA classes. Others also observed a more frequent detection of dnDSA against class II (77%) than against class I (38%) HLA antigens76. In this study, class I alloantibodies made up 42% of early DSA (< 6 months after transplantation) but were only 29% of late DSA (> 6 months after transplantation). Conversely, class II was 58% of early DSA and 71% of late DSA. Importantly, no difference on allograft survival was observed comparing patients with dnDSA according to HLA class.
Another important observation reported by several studies is the high incidence of anti‑DQ dnDSA. In the referred cohort by Wiebe at al.75, out of 44 patients with class II DSA, 31 had anti‑DQ, while 21 had anti‑DR DSA.
Additionally, when they analyzed donor‑recipient HLA‑DR and ‑DQ matching at epitope level, higher epitope mismatch was significantly associated with dnDSA emergence against the respective locus81. More recently, they expanded these observations showing that non‑adherence and higher epitope mismatch acted synergistically towards a higher risk of rejection or allograft loss82. Hence, the evaluation of patients adherence and epitope mismatch load are valuable tools in the identification of patients that could benefit from increased clinical, histologic and immunological surveillance.
Complement‑binding DSA
Modified SAB assays analyzing complement binding DSA ability (C1q‑ and C3d‑SAB) have been applied widely in the post‑transplant setting63. A large study examining the impact of C1q‑binding DSA on kidney transplantation, reported that C1q+ DSA, when present in the first year after transplantation were associated with a higher risk of allograft loss (HR=4.78, P<0.001)64.
These alloantibodies were also associated with an increased rate of AMR, a more extensive microvascular inflammation, and increased deposition of C4d within allograft capillaries. Others have demonstrated that presence of C3d‑binding DSA at the time of AMR diagnosis (HR=2.80, P=0.03) was an independent predictor of allograft loss65. More recently, Guidicelli et al.83 observed that C1q‑binding dnDSA were associated with allograft loss occurring rapidly after their appearance.
However, the long‑term persistence of C1q‑nonbinding dnDSA also led to lower allograft survival, though in a more protracted manner.
These results raised great expectations in the transplant community, that these modified SAB assays could allow the identification of patients with dnDSA at a higher risk of allograft failure, and in whom specific treatments could be appropriate. However, these observations were not confirmed by other groups (59, 66), so the task of pertinently adjudicating risk when a patient is diagnosed with dnDSA remains challenging.
Furthermore, some consider it advisable to perform histological evaluation when dnDSA are detected80, although the absence of an effective treatment for subclinical dnDSA hampers this recommendation.
Immunoglobulin subclasses
Characterization of IgG subclasses has been performed by a modified SAB assay in the research setting, since originally it only measured total IgG (pan‑IgG)84.
Lefaucheur et al.85 showed, in patients with DSA detected at 1‑year post‑transplant, that acute AMR was mainly driven by IgG3 DSA, whereas subclinical AMR by IgG4 DSA. Additionally, they demonstrated that IgG3 DSA was strongly and independently associated with allograft failure. These results provide clues about the pathogenicity of certain DSA and the immune response ensued by them. Still, the complexity of studying IgG subclasses constrains the possibility of its application in the clinical setting.
CONCLUSIONS
Laboratory assays for the study of HLA alloantibodies are crucial in kidney transplantation and have evolved greatly, from cell‑based crossmatches to SPI. Although most information added by these new assays is valuable, its clinical relevance is not always unequivocal, so they should be discussed in an integrated manner between HLA laboratory experts and clinicians, in order to fully encompass each tests sensitivity and limitations. As such, the stratification of preformed DSA risk demands this concerted effort, to secure a correct identification of DSA and a meaningful assessment of the clinical implications pertained by them. While the analysis of DSA characteristics, as MFI or complement‑binding ability, gives valuable information about the risk involved, it should be accompanied by a thorough evaluation of clinical data and the results of cell‑based crossmatches. In the post‑transplant setting, dnDSA screening should be individualized according to the immunological risk of each patient, determined not only at transplant (e.g., sensitization status, epitope mismatch load), but also according to significant clinical events (e.g., rejection episodes, documented non‑adherence) observed afterwards. The emergence of dnDSA is, by itself, a risk factor for adverse outcomes, still a comprehensive alloantibody characterization, as complement‑binding ability, may allow in each case a more granular analysis of the risk posed by dnDSA, particularly if concurrent histological evaluation is obtained.
References
1. Zhang R. Donor‑specific antibodies in kidney transplant recipients. Clin J Am Soc Nephrol. 2018 Jan 6;13(1):182‑192. [ Links ]
2. Orandi BJ, Luo X, Massie AB, Garonzik‑Wang JM, Lonze BE, Ahmed R, et al. Survival benefit with kidney transplants from HLA‑incompatible live donors. N Engl J Med. 2016;374(10):940‑50. [ Links ]
3. Terasaki PI, Mcclelland JD. Microdroplet Assay of Human Serum Cytotoxins. Nature. 1964;204:998‑1000. [ Links ]
4. Mullis KB. Target amplification for DNA analysis by the polymerase chain reaction. Ann Biol Clin. 1990;48(8):579‑82. [ Links ]
5. Olerup O, Zetterquist H. HLA‑DR typing by PCR amplification with sequence‑specific primers (PCR‑SSP) in 2 hours: an alternative to serological DR typing in clinical practice including donor‑recipient matching in cadaveric transplantation. Tissue Antigens. 1992;39(5):225‑35. [ Links ]
6. Saiki RK, Walsh PS, Levenson CH, Erlich HA. Genetic analysis of amplified DNA with immobilized sequence‑specific oligonucleotide probes. Proc Natl Acad Sci U S A. 1989;86(16):6230‑4. [ Links ]
7. Wu J, Griffith BB, Bassinger S, Moehlenkamp C, Brodie SG, Wu Y, et al. Strategies for unambiguous detection of allelic heterozygosity via direct DNA sequencing of PCR products: application to the HLA DRB1 locus. Mol Diagn. 1996;1(2):89‑98. [ Links ]
8. Gabriel C, Fürst D, Faé I, Wenda S, Zollikofer C, Mytilineos J, et al. HLA typing by next‑generation sequencing getting closer to reality. Tissue Antigens. 2014;83(2):65‑75. [ Links ]
9. Zachary AA, Leffell MS. HLA mismatching strategies for solid organ transplantation a balancing act. Front Immunol. 2016;7:575. [ Links ]
10. Opelz G, Döhler B. HLA matching and kidney transplantation: beyond graft survival. Clin Transpl. 2013:121‑6. [ Links ]
11. Duquesnoy RJ, Gebel HM, Woodle ES, Nickerson P, Baxter‑Lowe LA, Bray RA, et al. High‑resolution HLA typing for sensitized patients: advances in medicine and science require us to challenge existing paradigms. Am J Transplant. 2015;15(10):2780‑1. [ Links ]
12. Cecka JM, Reed EF, Zachary AA. HLA high‑resolution typing for sensitized patients: a solution in search of a problem? Am J Transplant. 2015;15(4):855‑6. [ Links ]
13. Duquesnoy RJ. Are we ready for epitope‑based HLA matching in clinical organ transplantation? Transplantation. 2017;101(8):1755‑65. [ Links ]
14. Amrouche L, Aubert O, Suberbielle C, Rabant M, Van Huyen JD, Martinez F, et al. Long‑term outcomes of kidney transplantation in patients with high levels of preformed DSA: the necker high‑risk transplant program. Transplantation. 2017;101(10):2440‑8. [ Links ]
15. Tafulo S, Malheiro J, Dias L, Mendes C, Osório E, Martins LS, et al. Low transplantability of 0 blood group and highly sensitized candidates in the Portuguese kidney allocation algorithm: quantifying an old problem in search of new solutions. HLA. 2016;88(5):232‑8. [ Links ]
16. Lopes D, Barra T, Malheiro J, Tafulo S, Martins L, Almeida M, et al. Effect of different sensitization events on HLA alloimmunization in kidney transplantation candidates. Transplant Proc. 2015;47(4):894‑7. [ Links ]
17. Böyum A. Isolation of mononuclear cells and granulocytes from human blood. Isolation of monuclear cells by one centrifugation, and of granulocytes by combining centrifugation and sedimentation at 1 g. Scand J Clin Lab Invest Suppl. 1968;97:77‑89. [ Links ]
18. Liwski RS, Greenshields AL, Conrad DM, Murphey C, Bray RA, Neumann J, et al. Rapid optimized flow cytometric crossmatch (FCXM) assays: The Halifax and Halifaster protocols. Hum Immunol. 2018 Jan;79(1):28‑38. [ Links ]
19. Terasaki PI, Mickey MR, Cecka M, Iwaki Y, Cicciarelli J. Clinical kidney transplants, 1988. Immunol Lett. 1989;21(1):33‑8. [ Links ]
20. Gebel HM, Bray RA, Nickerson P. Pre‑transplant assessment of donor‑reactive, HLA‑specific antibodies in renal transplantation: contraindication vs. risk. Am J Transplant. 2003;3(12):1488‑500. [ Links ]
21. Amos DB, Bashir H, Boyle W, MacQueen M, Tiilikainen A. A simple micro cytotoxicity test. Transplantation. 1969;7(3):220‑3. [ Links ]
22. Fuller TC, Cosimi AB, Russell PS. Use of an antiglobulin‑ATG reagent for detection of low levels of alloantibody‑improvement of allograft survival in presensitized recipients. Transplant Proc. 1978;10(2):463‑6. [ Links ]
23. Tellis VA, Matas AJ, Senitzer D, Louis P, Glicklich D, Soberman R, et al. Successful transplantation after conversion of a positive crossmatch to negative by dissociation of IgM antibody. Transplantation. 1989;47(1):127‑9. [ Links ]
24. Cross DE, Greiner R, Whittier FC. Importance of the autocontrol crossmatch in human renal transplantation. Transplantation. 1976;21(4):307‑11. [ Links ]
25. Scornik JC, Brunson ME, Schaub B, Howard RJ, Pfaff WW. The crossmatch in renal transplantation. Evaluation of flow cytometry as a replacement for standard cytotoxicity. Transplantation. 1994;57(4):621‑5. [ Links ]
26. Garovoy M, Rheinschmidt M, Bigos M, Perkins H, Colombe B, Feduska N, et al. Flow cytometry analysis: a high tecnhology crossmatch tecnhique facilitating transplantation. Transplant. Proc; 1983.1939‑44. [ Links ]
27. Bray RA, Lebeck LK, Gebel HM. The flow cytometric crossmatch. Dual‑color analysis of T cell and B cell reactivities. Transplantation. 1989;48(5):834‑40. [ Links ]
28. Robson A, Martin S. T and B cell crossmatching using three‑colour flow cytometry. Transplant Immunology. 1996; 203‑8. [ Links ]
29. Lobo PI, Spencer CE, Stevenson WC, McCullough C, Pruett TL. The use of pronase‑digested human leukocytes to improve specificity of the flow cytometric crossmatch. Transpl Int. 1995;8(6):472‑80. [ Links ]
30. Lobo PI, Isaacs RB, Spencer CE, Pruett TL, Sanfey HA, Sawyer RG, et al. Improved specificity and sensitivity when using pronase‑digested lymphocytes to perform flow‑cytometric crossmatch prior to renal transplantation. Transpl Int. 2002;15(11):563‑9. [ Links ]
31. Alheim M, Wennberg L, Wikström AC. Pronase independent flow cytometry crossmatching of rituximab treated patients. Hum Immunol. 2017; [ Links ]
32. Doxiadis I, Westhoff U, Grosse‑Wilde H. Quantification of soluble HLA class I gene products by an enzyme linked immunosorbent assay. Blut. 1989;59(5):449‑54. [ Links ]
33. Pei R, Wang G, Tarsitani C, Rojo S, Chen T, Takemura S, et al. Simultaneous HLA Class I and Class II antibodies screening with flow cytometry. Hum Immunol. 1998;59(5):313‑22. [ Links ]
34. Pei R, Lee J, Chen T, Rojo S, Terasaki PI. Flow cytometric detection of HLA antibodies using a spectrum of microbeads. Hum Immunol. 1999;60(12):1293‑302. [ Links ]
35. Pei R, Lee JH, Shih NJ, Chen M, Terasaki PI. Single human leukocyte antigen flow cytometry beads for accurate identification of human leukocyte antigen antibody specificities. Transplantation. 2003;75(1):43‑9. [ Links ]
36. Reed EF, Rao P, Zhang Z, Gebel H, Bray RA, Guleria I, et al. Comprehensive assessment and standardization of solid phase multiplex‑bead arrays for the detection of antibodies to HLA‑drilling down on key sources of variation. Am J Transplant. 2013;13(11):3050‑1. [ Links ]
37. Chen G, Sequeira F, Tyan DB. Novel C1q assay reveals a clinically relevant subset of human leukocyte antigen antibodies independent of immunoglobulin G strength on single antigen beads. Hum Immunol. 2011;72(10):849‑58. [ Links ]
38. Stegall MD, Chedid MF, Cornell LD. The role of complement in antibody‑mediated rejection in kidney transplantation. Nat Rev Nephrol. 2012;8(11):670‑8. [ Links ]
39. Diebolder CA, Beurskens FJ, de Jong RN, Koning RI, Strumane K, Lindorfer MA, et al. Complement is activated by IgG hexamers assembled at the cell surface. Science. 2014;343(6176):1260‑3. [ Links ]
40. Tambur AR, Herrera ND, Haarberg KM, Cusick MF, Gordon RA, Leventhal JR, et al. Assessing antibody strength: comparison of MFI, C1q, and titer information. Am J Transplant. 2015;15(9):2421‑30. [ Links ]
41. American Society for H, Immunogenetics, Hahn AB, Land GA, Strothman RM. ASHI laboratory manual. [Mount Laurel, N.J.]: American Society for Histocompatibility and Immunogenetics; 2000. [ Links ]
42. Cai J, Terasaki PI, Anderson N, Lachmann N, Schönemann C. Intact HLA not beta2m‑free heavy chain‑specific HLA class I antibodies are predictive of graft failure. Transplantation. 2009;88(2):226‑30. [ Links ]
43. Nair V, Sawinski D, Akalin E, Friedlander R, Ebcioglu Z, Sehgal V, et al. Effect of high‑dose intravenous immunoglobulin on anti‑HLA antibodies in sensitized kidney transplant candidates. Clin Transplant. 2012;26(3):E261‑8. [ Links ]
44. Masson E, Devillard N, Chabod J, Yannaraki M, Thevenin C, Tiberghien P, et al. Misleading de novo detection of serum anti‑HLA A3 antibodies in kidney recipients having received ATG before transplantation. Hum Immunol. 2010;71(2):170‑5. [ Links ]
45. Tait BD, Süsal C, Gebel HM, Nickerson PW, Zachary AA, Claas FH, et al. Consensus guidelines on the testing and clinical management issues associated with HLA and non‑HLA antibodies in transplantation. Transplantation. 2013;95(1):19‑47. [ Links ]
46. Gombos P, Opelz G, Scherer S, Morath C, Zeier M, Schemmer P, et al. Influence of test technique on sensitization status of patients on the kidney transplant waiting list. Am J Transplant. 2013;13(8):2075‑82. [ Links ]
47. Zachary AA, Braun WE. Calculation of a predictive value for transplantation. Transplantation.1985;39(3):316‑8. [ Links ]
48. Cecka JM. Calculated PRA (CPRA): the new measure of sensitization for transplant candidates. Am J Transplant. 2010;10(1):26‑9. [ Links ]
49. Baxter‑Lowe LA, Kucheryavaya A, Tyan D, Reinsmoen N. CPRA for allocation of kidneys in the US: More candidates ≥98% CPRA, lower positive crossmatch rates and improved transplant rates for sensitized patients. Hum Immunol. 2016;77(5):395‑402. [ Links ]
50. Montgomery RA, Lonze BE, King KE, Kraus ES, Kucirka LM, Locke JE, et al. Desensitization in HLA‑incompatible kidney recipients and survival. N Engl J Med. 2011;365(4):318‑26. [ Links ]
51. Vo AA, Jordan SC. Benefits, efficacy, cost‑effectiveness and infectious complications in transplant patients desensitized with intravenous immunoglobulin and anti‑CD20 therapy. Clin Exp Immunol. 2014;178(1):48‑51. [ Links ]
52. Kannabhiran D, Lee J, Schwartz JE, Friedlander R, Aull M, Muthukumar T, et al. Characteristics of circulating donor human leukocyte antigen‑specific immunoglobulin g antibodies predictive of acute antibody‑mediated rejection and kidney allograft failure. Transplantation. 2015;99(6):1156‑64. [ Links ]
53. Zecher D, Bach C, Staudner C, Boger CA, Bergler T, Banas B, et al. Characteristics of donor‑specific anti‑HLA antibodies and outcome in renal transplant patients treated with a standardized induction regimen. Nephrol Dial Transplant. 2017;32(4):730‑7. [ Links ]
54. Schwaiger E, Eskandary F, Kozakowski N, Bond G, Kikic Z, Yoo D, et al. Deceased donor kidney transplantation across donor‑specific antibody barriers: predictors of antibody‑mediated rejection. Nephrol Dial Transplant. 2016;31(8):1342‑51. [ Links ]
55. Malheiro J, Tafulo S, Dias L, Martins LS, Fonseca I, Beirao I, et al. Analysis of preformed donor‑specific anti‑HLA antibodies characteristics for prediction of antibody‑mediated rejection in kidney transplantation. Transpl Immunol. 2015;32(2):66‑71. [ Links ]
56. Salvade I, Aubert V, Venetz JP, Golshayan D, Saouli AC, Matter M, et al. Clinically‑relevant threshold of preformed donor‑specific anti‑HLA antibodies in kidney transplantation. Hum Immunol. 2016;77(6):483‑9. [ Links ]
57. Vo AA, Sinha A, Haas M, Choi J, Mirocha J, Kahwaji J, et al. Factors predicting risk for antibody‑mediated rejection and graft loss in highly human leukocyte antigen sensitized patients transplanted after fesensitization. Transplantation. 2015;99(7):1423‑30. [ Links ]
58. Schinstock CA, Gandhi MJ, Stegall MD. Interpreting anti‑HLA antibody testing data: a practical guide for physicians. Transplantation. 2016;100(8):1619‑28. [ Links ]
59. Wiebe C, Gareau AJ, Pochinco D, Gibson IW, Ho J, Birk PE, et al. Evaluation of C1q status and titer of de novo donor‑specific antibodies as predictors of allograft survival. Am J Transplant. 2017;17(3):703‑11. [ Links ]
60. McKenna RM, Takemoto SK, Terasaki PI. Anti‑HLA antibodies after solid organ transplantation. Transplantation. 2000;69(3):319‑26. [ Links ]
61. Bachelet T, Martinez C, Del Bello A, Couzi L, Kejji S, Guidicelli G, et al. Deleterious impact of donor‑specific anti‑HLA antibodies toward HLA‑Cw and HLA‑DP in kidney transplantation. Transplantation. 2016;100(1):159‑66. [ Links ]
62. Carta P, Di Maria L, Caroti L, Buti E, Antognoli G, Minetti EE. Anti‑human leukocyte antigen DQ antibodies in renal transplantation: Are we underestimating the most frequent donor specific alloantibodies? Transplant Rev (Orlando). 2015;29(3):135‑8. [ Links ]
63. Bohmig GA, Kikic Z, Wahrmann M, Eskandary F, Aliabadi AZ, Zlabinger GJ, et al. Detection of alloantibody‑mediated complement activation: a diagnostic advance in monitoring kidney transplant rejection? Clin Biochem. 2016;49(4‑5): 394‑403. [ Links ]
64. Loupy A, Lefaucheur C, Vernerey D, Prugger C, Duong van Huyen JP, Mooney N, et al. Complement‑binding anti‑HLA antibodies and kidney‑allograft survival. N Engl J Med. 2013;369(13):1215‑26. [ Links ]
65. Sicard A, Ducreux S, Rabeyrin M, Couzi L, McGregor B, Badet L, et al. Detection of C3d‑binding donor‑specific anti‑HLA antibodies at diagnosis of humoral rejection predicts renal graft loss. J Am Soc Nephrol. 2015;26(2):457‑67.
66. Yell M, Muth BL, Kaufman DB, Djamali A, Ellis TM. C1q Binding activity of de novo donor‑specific HLA antibodies in renal transplant recipients with and without antibody‑mediated rejection. Transplantation. 2015;99(6):1151‑5. [ Links ]
67. Crespo M, Torio A, Mas V, Redondo D, Perez‑Saez MJ, Mir M, et al. Clinical relevance of pretransplant anti‑HLA donor‑specific antibodies: does C1q‑fixation matter? Transpl Immunol. 2013;29(1‑4): 28‑33.
68. Malheiro J, Tafulo S, Dias L, Martins S, Fonseca I, Beirao I, et al. Determining donor‑specific antibody C1q‑binding ability improves the prediction of antibody‑mediated rejection in human leucocyte antigen‑incompatible kidney transplantation. Transpl Int. 2017;30(4):347‑59. [ Links ]
69. Molina J, Navas A, Aguera ML, Rodelo‑Haad C, Alonso C, Rodriguez‑Benot A, et al. Impact of preformed donor‑specific anti‑human leukocyte antigen antibody C1q‑binding ability on kidney allograft outcome. Front Immunol. 2017;8:1310. [ Links ]
70. Schaub S, Honger G, Koller MT, Liwski R, Amico P. Determinants of C1q binding in the single antigen bead assay. Transplantation. 2014;98(4):387‑93. [ Links ]
71. Tait BD, Susal C, Gebel HM, Nickerson PW, Zachary AA, Claas FH, et al. Consensus guidelines on the testing and clinical management issues associated with HLA and non‑HLA antibodies in transplantation. Transplantation. 2013;95(1):19‑47. [ Links ]
72. Loupy A, Hill GS, Jordan SC. The impact of donor‑specific anti‑HLA antibodies on late kidney allograft failure. Nat Rev Nephrol. 2012;8(6):348‑57 [ Links ]
73. Sellares J, de Freitas DG, Mengel M, Reeve J, Einecke G, Sis B, et al. Understanding the causes of kidney transplant failure: the dominant role of antibody‑mediated rejection and nonadherence. Am J Transplant. 2012;12(2):388‑99. [ Links ]
74. Castro A, Malheiro J, Tafulo S, Dias L, Martins LS, Fonseca I, et al. Role of de novo donor‑specific anti‑HLA antibodies in kidney graft failure: a case‑control study. HLA. 2017;90(5):267‑75. [ Links ]
75. Wiebe C, Gibson IW, Blydt‑Hansen TD, Karpinski M, Ho J, Storsley LJ, et al. Evolution and clinical pathologic correlations of de novo donor‑specific HLA antibody post kidney transplant. Am J Transplant. 2012;12(5):1157‑67. [ Links ]
76. Everly MJ, Rebellato LM, Haisch CE, Ozawa M, Parker K, Briley KP, et al. Incidence and impact of de novo donor‑specific alloantibody in primary renal allografts. Transplantation. 2013;95(3):410‑7. [ Links ]
77. Malheiro J, Martins LS, Tafulo S, Dias L, Fonseca I, Beirão I, et al. Impact of de novo donor‐specific anti‐HLA antibodies on grafts outcomes in simultaneous pancreaskidney transplantation. Transplant International. 2016;29(2):173‑83. [ Links ]
78. Wiebe C, Gibson IW, Blydt‑Hansen TD, Pochinco D, Birk PE, Ho J, et al. Rates and determinants of progression to graft failure in kidney allograft recipients with de novo donor‑specific antibody. Am J Transplant. 2015;15(11):2921‑30. [ Links ]
79. Heilman RL, Nijim A, Desmarteau YM, Khamash H, Pando MJ, Smith ML, et al. De novo donor‑specific human leukocyte antigen antibodies early after kidney transplantation. Transplantation. 2014;98(12):1310‑5. [ Links ]
80. Schinstock CA, Cosio F, Cheungpasitporn W, Dadhania DM, Everly MJ, Samaniego‑Picota MD, et al. The value of protocol biopsies to identify patients with de novo donor‑specific antibody at high risk for allograft loss. Am J Transplant. 2017;17(6):1574‑84. [ Links ]
81. Wiebe C, Pochinco D, Blydt‑Hansen TD, Ho J, Birk PE, Karpinski M, et al. Class II HLA epitope matching‑A strategy to minimize de novo donor‑specific antibody development and improve outcomes. Am J Transplant. 2013;13(12):3114‑22. [ Links ]
82. Wiebe C, Nevins TE, Robiner WN, Thomas W, Matas AJ, Nickerson PW. The synergistic effect of class II HLA epitope‑mismatch and nonadherence on acute rejection and graft survival. Am J Transplant. 2015;15(8):2197‑202. [ Links ]
83. Guidicelli G, Guerville F, Lepreux S, Wiebe C, Thaunat O, Dubois V, et al. Non‑complement‑binding de novo donor‑specific anti‑HLA antibodies and kidney allograft survival. J Am Soc Nephrol. 2016;27(2):615‑25. [ Links ]
84. Freitas MC, Rebellato LM, Ozawa M, Nguyen A, Sasaki N, Everly M, et al. The role of immunoglobulin‑G subclasses and C1q in de novo HLA‑DQ donor‑specific antibody kidney transplantation outcomes. Transplantation. 2013;95(9):1113‑9. [ Links ]
85. Lefaucheur C, Viglietti D, Bentlejewski C, Duong van Huyen JP, Vernerey D, Aubert O, et al. IgG donor‑specific anti‑human HLA antibody subclasses and kidney allograft antibody‑mediated injury. J Am Soc Nephrol. 2016;27(1):293‑304. [ Links ]
Jorge Malheiro
Nephrology & Kidney Transplantation Department,
Centro Hospitalar do Porto, Hospital de Santo António,
Largo Prof. Abel Salazar 4099‑001
Porto, Portugal.
E‑mail: jjorgemalheiro@gmail.com
Disclosure of potential conflicts of interest: none declared
Received for publication: Dec 2, 2017
Accepted in revised form: Dec 15, 2017