INTRODUCTION
Health care industry is one of the service sectors with the highest impact on carbon footprint in the industrialized world.1-3 It is responsible for more than 4% of worldwide greenhouse gas emissions and similar fractions of toxic air pollutants, mainly caused by fossil fuel combustion.1,2 Health care footprint as a percentage of national emissions in Portugal is estimated to be 4.8%.4
Nephrology, and in particular dialysis, is considered one of the most “resource-intensive” fields of medicine.5,6This fact led to a position statement from the Italian Society of Nephrology on eco-dialysis and green nephrology, namely on ways in which the environmental impact of caring for patients with kidney diseases can be reduced.7
In fact, dialysis treatment consumes a significant amount of water and energy, with an important waste management effort. Those variables play a relevant role on the total cost of a dialysis session.
The number of dialysis patients remains stable in mature highincome countries and will continue to grow rapidly, especially in lowincome and middle- income countries, due to population ageing, increased prevalence of hypertension and diabetes mellitus, toxic environmental exposures and increase in the availability of dialysis.8
These facts tend to confirm that environmental burden of renal replacement therapy will remain present in the future. Of course, the dialysis treatment must be granted to patients, but the foreseeable rise in the consumption of supplies over the years to come and its growing environmental impact will require increased efforts to improve efficiency and reducing waste.
KIDNEY THERAPY (HEMODIALYSIS) AND WATER CONSUMPTION IN DIALYSIS UNITS
Water is an essential component of renal replacement therapy by dialysis. Water serves as a solvent to electrolytic concentrates for the preparation of dialysis fluids; therefore, the water treatment system (WTS) can be considered the kidney of any dialysis unit. Commonly a healthy adult is exposed (via ingestion) up to 14 L of water per week. Water proceeds through a selective barrier of the gastrointestinal tract and some toxic contaminants are removed by the renal function. In contrast, hemodialysis patients (undergoing 4-hour dialysis sessions, three times a week) are exposed to more than 360 L of dialysis fluid per week, this indicates that the blood of dialysis patients is exposed to 30 to 40 times more water than the general population. In addition, anuric dialysis patients are exposed for long periods of time and have considerable cumulative time exposure that needs to be considered in the risk assessment.9
Dialysis fluid and consequently water contaminants (even at micro or nanomolar concentrations) may pass through the non-selective dialyzer membrane and tend to accumulate overtime in dialysis patients due to the lack of excretory capacity by damaged kidneys.
Furthermore, the highly permeable membranes used today, as well as the increased prescription of more convective and high volumes therapies, rises the risk of contaminants load passing through the membrane and consequently into the blood.
Therefore, the chemical and microbiological quality of water used for dialysis is of the utmost importance if an additional health risk to hemodialysis patients is to be avoided.
Strict dialysis water and ultrapure dialysis fluids standards were established as a pre-requisite for online convective therapies (e.g., hemodiafiltration), improving biocompatibility of the dialysis system, reducing inflammation profile of the dialysis patients, enhancing the responsiveness to erythropoiesis-stimulating agents, and thus having the potential for improved survival.10-15
Reported water consumption for a single treatment varies from 400 L up to 500 L,16-18 which includes not only the direct water consumption for preparation of dialysis fluids during treatment, but also priming, rinse, disinfection, inter-sessional idle phases, and water consumed in the water treatment system (e.g., backwashing and/or regeneration cycles of pre-treatment devices). The reported water consumption values are estimations from the authors based on several assumptions, namely a low-efficiency reverse osmosis (RO) system (i.e., with a recovery rate of less than 70%). Based on these assumptions, the annual water consumption in a 75-dialysis patient unit would be higher than the typical volume of 2 Olympic swimming pools (i.e., 11 700 treatments, with a consumption of 500 L/Tx would approximately represent 5850 m³ of water consumed in one year).
The importance of the WTS efficiency, namely the RO recovery rate, in the overall water consumption per treatment, is well illustrated by Bendine et al.19 In this article, the authors described the impact of updating several WTS in NephroCare centers in France (replacing old RO devices by updated ones with a significant improvement in efficiency in terms of the proportion of rejected water - from 60% to 20%). After implementation of an updated WTS, water consumption dropped by 50%.
THE MAIN PILLARS OF WATER CONSUMPTION IN DIALYSIS UNITS
To better understand the water consumption dynamics in a dialysis unit the following pillars should be considered:
1. Water consumption related to WTS pre-treatment and hygiene
To keep the WTS under control, both from a hydraulic and water quality points of view, there is a need of water consumption. The pre-treatment devices (e.g., multimedia filters, softeners and activated carbon filters) need to perform regular backwashing and/or regeneration cycles. The RO and distribution system(s) need to be frequently rinsed or flushed and properly disinfected to guarantee that the dialysis water quality microbial outcomes mee the specified values for dialysis. ISO 2350020 specifies the following action levels for dialysis water: <50 colony forming units (CFU)/mL and 0.125 endotoxin units (EU)/mL. Important to mention that water consumption relate to WT pre-treatment and hygiene, is independent of the dialysis unit treatments dynamics. In other words, it can be considered as fixed water consumption. Therefore, when translating the water consumption per treatment, the relative weight will be higher in smaller units (i.e., with lower number of treatments), or diluted in larger ones (i.e., with higher number of treatments). The effort to keep the system under control, avoiding biofouling issues, is usually higher (i.e., involving extra water and energy consumption) when dialysis units have longer periods of time without treatments being performed (either due to the fact that the units are over dimensioned or with less than three shifts per day).
2. Water consumption related to reverse osmosis efficiency
A crucial point in terms of water consumption is the setting of the RO device. In a RO there are three important ratios to consider: the rejection rate, the recovery rate, and the concentration factor.
The rejection rate evaluates how effective the RO is at removing contaminants present in water. It is defined as:
Monitoring RO rejection trends allow the assessment of membrane performance (commonly used to initiate maintenance and servisse actions). Frequent membrane cleaning and disinfection procedures are pre-requisites for maintaining an acceptable performance and dialysis water quality outcomes.
Recovery rate represents the amount of water that is not sent to drain as concentrate, but rather collected as permeate. It is defined as:
A reverse osmosis unit with a recovery rate (commonly referred as yield) of 70% means that for a final production of 70 L of dialysis water, 100 L of water are consumed, therefore 30 L of concentrate will be discarded to waste.
The concentration factor is related to the RO system recovery. It is defined as:
Concentration factor = [1/(1 - recovery %)]
But increasing the recovery rate to ever higher values come with a cost: it raises the concentration of salts and contaminants in the concentrate stream and leads to a higher potential for scaling at the membrane surface. As the concentration increases, the solubility limits may be exceeded and solutes precipitate on the surface of the membrane as scale. This phenomenon is known as concentration polarization.
Even with the right flow dynamics (e.g., inducing a turbulent flow at the membrane surface), the recovery rate and concentration factor need to be fine-tuned according to the feed water profile, considering the concentration limits of potential scalants.
The WTS efficiency is strongly influenced by the system design and RO setup. A rejection rate of 95% and a recovery rate of over 70% are common figures in dialysis unit.9 However, some modern RO systems can operate at higher efficiency rates approaching a recovery rate of 85% (adjustments must take into account the inlet water quality profile).
3. Water consumption related to treatments
This involves the direct water consumption for preparation of dialysis fluids during treatment (i.e., water for dilution of concentrated hemodialysis solutions), but also priming, rinse, disinfection, and inter-sessional idle phases of the dialysis machines. The dialysis prescription (namely the dialysis fluid flow rates) plays an importante role in the dialysis fluid consumption and consequently the water consumption. Mesic et al21 compared individualized online hemodiafiltration (oHDF) and standard hemodialysis (HD), in terms of savings in dialysis fluid consumption and impact on dialysis dose. oHDF with automatic functions (i.e., autoflow function, a feature in a dialysis machine that couples the dialysis fluid flow rate to the blood flow rate) resulted in a lower dialysis fluid consumption (8.0% less) and in a slightly increased dialysis dose (Kt/V 3.5% higher) compared to HD.
4. Other water consumption sources
In a dialysis unit other water consumption factors exist, such as the so-called household water consumption (e.g., bathrooms, hands hygiene) and, in some cases laundry services. Usually, the relative weight of these consumptions in the overall clinic water consumption is low.
An example of the relative weight of the main pillars on the overall water consumption in a dialysis clinic is illustrated in Fig. 1. Example of the relative weight of the different pillars on the overall water consumption in a dialysis clinic (assumptions: dialysis unit with 75 patients, i.e., 11.700 Tx/year; 3 shifts/day; oHDF post-dilution with an autoflow factor of 1.2; average blood flow rates of 350mL/m; WTS with RO recovery rate of 75%; monthly R membranes and distribution system chemical disinfection and RO and distribution system flush during idle times - every two hours).
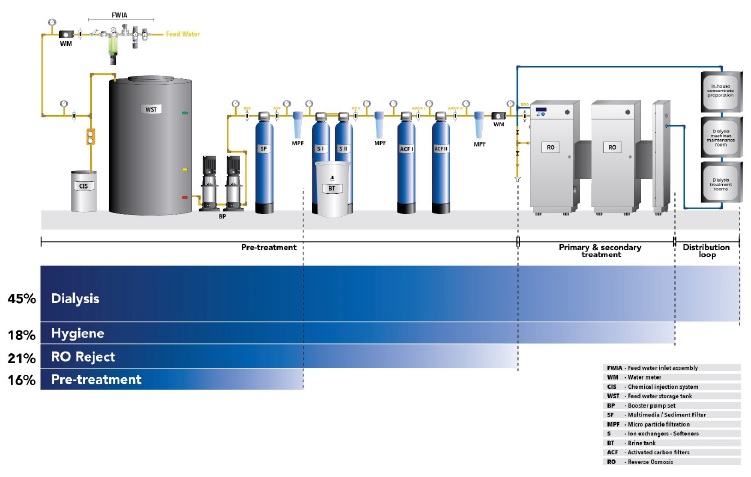
Figure 1 Example of the relative weight of the different pillars on the overall water consumption in a dialysis clinic (assumptions: dialysis unit with 75 patients, i.e., 11.700 Tx/year; 3 shifts/day; oHDF post-dilution with an autoflow factor of 1.2; average blood flow rates of 350 mL/m; WTS with RO recovery rate of 75%; monthly RO membranes and distribution system chemical disinfection and RO and distribution system flush during idle times - every two hours).
Word of caution when it comes to calculate the water consumption, either in terms of L/Tx or percentage. A dramatic change in any of the above-mentioned variables (i.e., assumptions) will have na impact in the ratio or percentage of water consumed, not necessarily meaning that a dialysis unit has lower or higher efficiency when compared to a different unit.
THE CHALLENGE OF THE INLET WATER QUALITY, IT´S IMPACT ON WTS PERFORMANCE AND EFFORT TO KEEP HYGIENE STATUS OF DIALYSIS WATER
The source of dialysis water is usually water meeting local drinking water standards, which is then submitted to a purification process that involves, among others, pressure driven membranes processes such as RO. However, when the water reaches the dialysis unit it has already passed through the building piping system, possibly changing the microbial profile. This situation becomes more critical as water passes through the pre-treatment system. Microbiological findings after softeners and carbon filters are up to 5000 CFU/mL for bacteria, 100 CFU/mL for fungi and 5-20 EU/mL.22
The RO membrane fouling phenomenon, because of the number of variations it can take, is very complex. Four main categories of fouling can be distinguished: particle / colloidal (e.g., deposition of clay, silt, silica), organic (e.g., deposition of dissolved humic acids, oil and grease), crystalline (mineral scaling), and microbiological (biofouling, adhesion and accumulation of microorganisms, forming biofilms).
The first three types of fouling can generally be controlled by reduction of foulant concentration in the water phase, according to the foulant removal rate. Biofouling, however, is hard to control by simply reducing the number of microorganisms in the water phase only, although this is the common approach at present for biofouling control (see Fig. 2).23
Because microorganisms are ubiquitous in technical systems and because disinfection neither leads to sterility, nor can be maintained over a long period of time, microorganisms will always invade and colonize the system. Especially in low nutrient systems, adhesion seems to be the strategy of bacteria to survive,23,24 forming biofilms capable of scavenging nutrients in very low concentration in the feed water. An effective prevention of microbial growth in membrane systems is achieved when a continuous, sufficiently disinfectant concentration is maintained. This, however, cannot be viewed as a possible solution in dialysis units.
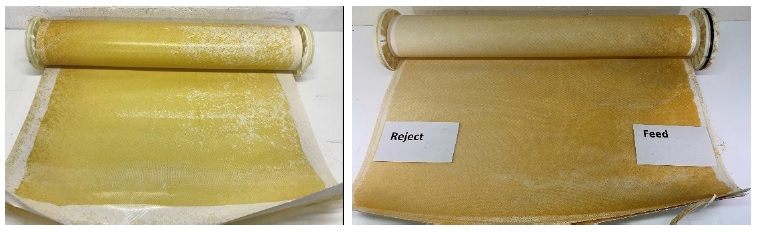
Figure 2 Images of biological foulant on autopsied reverse osmosis membranes (with permission from Avista|Centre of Excellence Kurita UK Ltd).
As stated in ISO 23500,20 a certain amount of biofilm formation in dialysis water systems is considered to be unavoidable. When the level of biofilm increases in such a way that the action levels for microorganisms and endotoxins in dialysis water are routinely exceeded, the operation of the system is compromised from a medical and technical point of view. The key to avoiding biofouling is to minimize biofilm development. The extent of biofilm growth is dependente on the availability of nutrients. Classic biocidal approaches usually do not limit nutrient availability. In fact, some biocides increase nutriente availability by oxidizing recalcitrant organics and making them more bioavailable.25
FROM A REACTIVE TO A PROPHYLACTIC APPROACH… IMPROVING THE RO INLET WATER QUALITY
The main purification step of a dialysis water treatment system is the reverse osmosis (RO). Fouling of reverse osmosis membranes remains a major issue and all efforts should be made to reduce it, to ensure a constant output. Fouling will cause an increase in the feed pressure, which can only be addressed by increasing the frequency of cleaning and reducing the system efficiency in terms of water and energy consumption. Naturally occurring waterborne filamentous fungi, yeasts, bacteria and fragments may contaminate dialysis water and, consequently, dialysis fluids.
To maintain the dialysis water treatment system under proper conditions and fulfilling the quality standards there is a need of a significant effort, namely in terms of cleaning and disinfection procedures of the RO and distribution systems.
It seems that the ideal situation to avoid the typical reactive approach of cleaning and disinfection procedures of RO and distribution system, would be to change the RO inlet water quality profile. Natural organic matter (NOM), biological (including bacteria and bacterial fragments), and colloidal scaling act as precursors of biofilm formation.
Ultrafiltration (UF) and microfiltration (MF) are considered as the best pre-treatment of feed water entering the reverse osmosis system to minimize membrane fouling.26,27
Lucena et al (under preparation) are presently studying the impact of a UF system as a pre-treatment step of a dialysis RO system, in terms of water quality outcomes, water and energy consumption, and disinfection frequency (see Fig. 3).
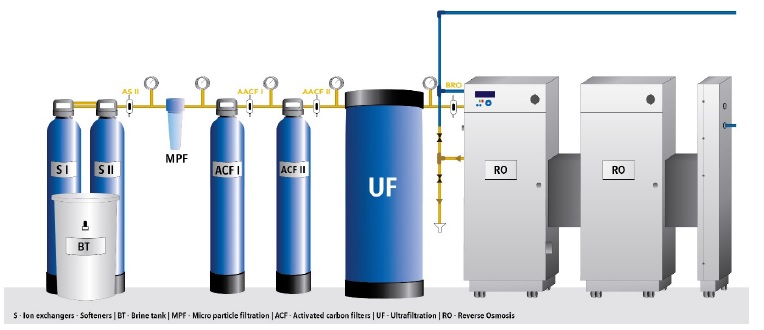
Figure 3 Schematic representation of a UF system as RO pre-treatment, changing the inlet water quality profile.
It is expected that the installation of a UF system as RO pre-treatment will have an impact on dialysis water quality, RO membrane organic, particle / colloidal, and microbiological fouling phenomenon,27 as well as an increase of RO membranes lifetime, leading to an overall positive effect on operating costs and sustainability key performance indicators (KPI) such as water consumption.
COMPREHENSIVE WATER SAVING STRATEGIES AND WATER REUSE
Taking the motto that one cannot manage what cannot be measured, estimation of water consumption based on invoices shall be replaced by real measurements. It is advised that a dialysis unit has, at least, the following water meters properly installed: inlet of the dialysis unit, inlet of the WTS, inlet of RO. This configuration would allow the unit to implement a monitoring system, with specific water consumption KPI. Monitoring can be a powerful tool and identify unexpected losses, possible water saving actions based on the presente consumption and to evaluate the effectiveness of the corrective/preventive actions.
A well designed WTS system (i.e., not over dimensioned) with a clear focus on increasing efficiency is mandatory if one wants to decrease the carbon footprint. Regarding the WTS pre-treatment devices (e.g., multimedia filters, softeners, carbon filters), the backwashing/regeneration cycles shall be performed only when needed (backwashing should be carefully planned by consume
more than 1000 L of water per backwashing and/or regeneration cycle. This water is usually discarded to the drain. The correct adjustment and particularly, the frequency of the backwashing cycles, may deliver higher savings. Reducing the frequency from daily to alternate days could save more than 600 000 L of water/year.9
As already stated, the RO setup, namely in terms of rejection/recovery rates, has a major impact, both in terms of water quality and efficiency. Whenever possible, the adjustment/increment of the recovery rate of the RO can bring up to a saving of more than 35 L of water per treatment. In a dialysis unit, where 15 000 treatments are performed annually, this adds up to a saving of more than 500 000 L of water/year.9 Some RO devices have a standby mode function (switch-on/off times). An automated switch-on feature will reduce unnecessary running times. “Switch-on times” can be set in such a way that the system starts producing water just before the dialysis shift starts. During the standby time, e.g., during night, the system should have a feature which allows a periodic running and water recirculation to avoid stagnation, but without working longer than necessary. Usually, a few minutes every two hours is adequate.9
The pre-treatment water quality (i.e., RO inlet) shall not be underestimated due to the impact on hygiene effort, namely the rinse/flush and disinfection procedures to keep WTS under control and to minimize biofouling issues.
The dialysis treatment requires the use of a dialysis concentrate that is most frequently distributed in 5 L bags or canisters. This represents the quantity, which is needed the for a single treatment, leading to an important carbon footprint (related with the transportation of the canisters). Further, large resources are needed for the disposal of plastic containers which are left at the end of the treatments.28 Keeping in mind that most of the canister content is water and that a source of dialysis water is available in each dialysis unit, it is worth considering an in-house concentrate preparation. In this case, only the active (dry or highly concentrated) components are carried to the dialysis unit. This approach has several advantages, namely regarding the cost of logistics and to environmental impacts, because no water is transported. In addition, the centralized distribution of concentrates allows the use of only the required quantity, without waste of unused concentrate solutions as it may happen with canisters.
Optimization of dialysis fluid flow rate (Qd) has a dramatic impact in terms of water consumption related with the dialysis treatments.
A very effective way to improve water consumption efficiency is the use of dialysis machines with an automatic adjustment of the dialysis fluid flow to the effective blood flow to ensure an optimal flow ratio. Dialysate regeneration system had its heydays in the eighties before falling in disuse due to greater technical complexity, electrolyte instability and limited efficiency.29 Today, dialysate regeneration system is retaking shape due to the availability of more efficient and compact sorbent cartridges, better mastered technology and most importantly the revived interest triggered by wearable artificial kidneys. Such sorbent technology offers currently the most attractive option to reduce water consumption in dialysis, insofar it permits to suppress water treatment system but also to minimize dialysate production, leading thus water consumption to less than 10 L per session.30
Collection of the reverse osmosis concentrate, and the water used in the backwashing of filters, for other purposes inside the dialysis unit, such as toilet flushing, will have an impact on the overall water consumption.16
Tarras et al31 proposed an algorithm strategy on possible reuse applications of hemodialysis reject water based on its characteristics.
In this algorithm, sodium adsorption ratio (SAR) assessment is suggested when total dissolved solids < 1.000 mg/L. A SAR value below.9 is considered safe for most gardening or lawn irrigation situations, while levels above 9 can cause severe problems in some soil typesover time.
Some authors reported on experiences about the reuse of reverse osmosis reject water for aquaponics and horticulture, suggesting that reject water can be reused to promote water conservation with encouraging results.32
The amount of water consumed should be regularly monitored and any leaks or misuse that contribute to excessive water consumption should be detected as soon as possible. The illustration below (Fig. 4) summarizes possible actions that can be taken to reduce the water consumption.
CONCLUSION
In summary, a more environmentally friendly dialysis can be influenced not only by the optimization of the current technology and the availability of new technology but also by the adoption of a conscious and coherent behavior of all persons involved in the daily routine of a dialysis unit.