Serviços Personalizados
Journal
Artigo
Indicadores
Links relacionados
Compartilhar
Portugaliae Electrochimica Acta
versão impressa ISSN 0872-1904
Port. Electrochim. Acta vol.34 no.5 Coimbra set. 2016
https://doi.org/10.4152/pea.201605321
Green Electrochemistry - A Versatile Tool in Green Synthesis: an Overview
Susai Rajendrana,* , R. Joseph Rathishb , S. Santhana Prabhab and A. Anandanc
a Research Centre, Department of Chemistry, RVS School of Engineering and Technology, Dindigul-624 005, Tamilnadu, India
b PSNA College of Engineering and Technology, Dindigul, India
c SKV Higher Secondary School, Kandampalayam-637201, India
Abstract
Green is attractive and beautiful. Green chemistry has attracted scientists and researchers from various fields. Electrolysis is considered as green electrochemistry, because electrochemical process can be stopped and controlled at any time and at any stage of the reaction. Usually water is used as the solvent. Corrosive acids are not used. Toxic chemicals are not involved. The main components of an electrolysis process are anode, cathode and electrolyte. By using suitable anodes, cathodes and medium, electrolysis has been applied in various fields. Electrolysis has been used to decolourise dyes from effluents of textile industries. The decolourisation efficiencies of various anodes, such as platinised-titanium, mild steel and aluminium in various electrolytic media, such as well water and sea water, have been evaluated and compared. The role of positive chlorine in the decolourisation process has been established. Electrolysis, in presence of a suitable reducing agent, has also been used to synthesize nanoparticles. Copper nanoparticles and silver nanoparticles have been produced by making use of reducing agents, such as sodium potassium tartrate and trisodium citrate. Various plants extracts have also been used as reducing agents. The nanoparticles synthesized by green methods have been characterized by UV-Visible absorption spectroscopy and fluorescence spectroscopy. The surface morphology of these nanoparticles has been characterized by SEM and EDS. Blue pigment that can be used in paint industry has been synthesized by green electrolysis. For this purpose, waste mild steel rod has been used, and graphite has been used as anode. The blue pigment prepared has been characterized by UV-Visible absorption spectroscopy and fluorescence spectroscopy. The surface morphology of these nanoparticles has been characterized by SEM and EDS. The blue pigment prepared is found to be in the nano range. This can be used in paint industry and also in the field of nano biosensors. By green electrolysis method, Hofmann rearrangement has been successfully effected, benzamide being the starting material. The product obtained has been diazotised and coupled with α-napthol and β-napthol to give dyes. The dyes have been characterized by UV-Visible absorption spectroscopy and fluorescence spectroscopy. Polyaniline has also been synthesized by green electrolysis process, without using expensive oxidizing agents, but using positive chloride ions generated in-situ during electrolysis, in the presence of sodium chloride solution as electrolyte. The polyaniline produced has been characterized by UV-Visible absorption spectroscopy, fluorescence spectroscopy and FTIR spectra.
Keywords: Green chemistry, green electrochemistry, green synthesis, nanoparticles, blue pigment, decolourisation, Hoffman rearrangement, polyaniline.
Introduction
Electrochemistry deals with chemical changes brought out by electricity, and electricity generated by chemical changes. Electron is the king of Electrochemistry. It rules electrochemistry. Electricity is generated, and chemical reactions take place, due to the movement of electrons from one source to another. Electron generation occurs at one place; the electron moves through a medium, and reaches a particular site. The electron is generated at the anode and it moves through the electrical circuit outside the solution system. It enters the solution system through the cathode and travels towards the anode through a medium which is called an electrolyte (Fig. 1).
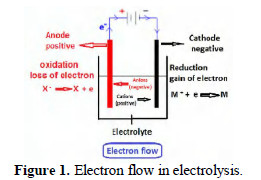
During this process many chemical reactions take place. The important components of this process are a source of AC electricity, a rectifier to produce DC current, an anode, a cathode and an electrolyte solution. An ammeter is connected in series, and a voltmeter in parallel. This experimental setup can be used for a process called electrolysis (Fig. 2).
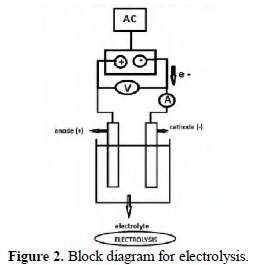
By selecting a variety of anodes, cathodes, electrolytic medium, solvent, potential difference (volt) and current density, many wonders can be done in the undivided cell. When an aqueous solution of sodium chloride is electrolyzed, Cl+ is generated at anode [1]. Various other species are also produced as per the views of many other scientists. Cl+ can be used to bring about variety of chemical reactions.
Dyes from textile effluents can be removed by chemical method [2], bio chemical method [3] and electrochemical method [4].
Nano particles can be generated by various methods, by reducing the particular metal ions, by using suitable reducing agents and capping agents [5].Hofmann rearrangement has been conventionally effected by making use of acid amides and hypo halites to produce primary amines [6]. Polyaniline has been produced from aniline, by making use of a variety of oxidizing agents [7]. In the old days our environment was healthy and safe, because there was less industrialization, and there was no deforestation, but rather continuous forestation. Because of the development of science and technology and the greediness of mankind, our environment has been polluted. To protect the nature, principles of green chemistry have been formulated (Figs. 3 and 4).
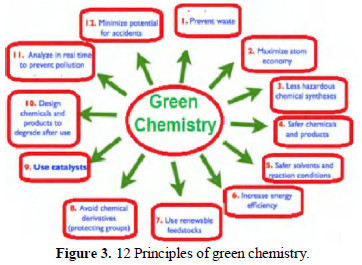
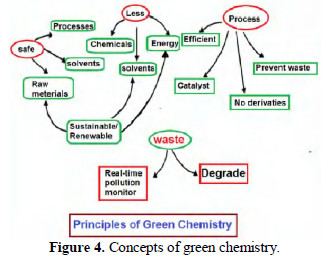
These principles emphasize that safe processes, raw materials and solvents must be used.
The use of solvents, energy and chemicals must be lesser. Any green process should be efficient, prevent waste and make use of catalysts. Raw materials, solvents and energy should be sustainable and renewable. The present work is undertaken to employ green electrolysis processes in the following events:
• Decolourisation of dyes;
• Production of a blue pigment from waste rusted iron rods;
• Electrochemical synthesis of silver nano particles and copper nano particles;
• To effect Hofmann rearrangement, there was an electrochemical start from benzamide and dyes were produced from the product obtained from electrolysis;
• To produce poly aniline electrochemically Cl+ was used as oxidizing agent.
In all the cases water is used as solvent; non toxic chemicals are used; less expensive reagents are used. Ordinary sodium chloride, even commercial sodium chloride, can be used to produce Cl+, which acts as a wonderful oxidizing agent. Apart from platinised titanium anode, other less expensive electrodes such as aluminium, mild steel, graphite, copper and silver are used. This process is an electrochemical process. Hence, the reaction can be started and stopped at will. No hazardous side products are produced. The environments are very safe. Wealth is produced even from waste materials such as waste iron rods. Natural product extracts have been used, in some cases as reducing agents, as in the case of production of silver nano particles, by reducing the silver ions produced electrochemically/chemically by neem extract.
Experimental
Electrolysis
Electrolysis was carried out in an undivided cell. Graphite was used as cathode. The anode was platinised titanium or aluminium or mild steel or copper. The solvent was water (well water) or double distilled water or sea water. In some cases sodium chloride was also added. For synthesis of nano particles double distilled water was used and the chemicals were very pure. For other cases, chemicals readily available were used. The following experiments were carried out:
• Electrochemical decolourisation of dyes;
• Electrochemical synthesis of blue pigment;
• Electrochemical synthesis of silver nano particles and copper nano particles;
• Electrochemical Hofmann rearrangement;
• Electrochemical synthesis of poly aniline.
Results and discussion
In this section, the following are discussed:
• Electrochemical decolourisation of dyes;
• Electrochemical synthesis of blue pigment;
• Electrochemical synthesis of silver nano particles and copper nano particles;
• Electrochemical Hofmann rearrangement;
• Electrochemical synthesis of poly aniline.
Electrochemical decolourisation of dye
A blue dye ''Blue ARDE dye'' used in dyeing industry has been decolourised in the present study. An aqueous solution of this dye (2%) has been used in this electrolysis process. Graphite was used as cathode, mild steel or platinised titanium were used as anode. Sodium chloride solution was used as electrolyte. When the dye solution was electrolysed, decolourisation took place effectively (Fig. 5).
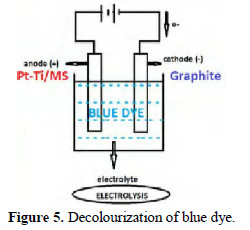
Optical densities were measured before and after decolourisation. Decolourisation efficiency (DE) was calculated using the equation:

where OD1 and OD2 are optical densities before and after decolourisation, respectively.
he results are given in Table 1.
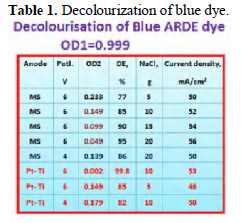
It is observed that platinised titanium offered better decolourisation efficiency than mild steel. As the concentration of sodium chloride increases, DE also increases, because in presence of higher concentration of NaCl, more Cl+ is produced and hence, more dye molecules were decolourised. For a given concentration of sodium chloride, as the applied voltage increases, DE also increases, since the flow of electrons increases as the potential difference increases. For a given concentration of NaCl and a given voltage, platinised titanium offered better DE than mild steel.
Mechanism of decolourisation
When sodium chloride solution is electrolyzed, positive chloride (Cl+) is produced [1]. This converts coloured compounds into colourless products (Fig. 6).
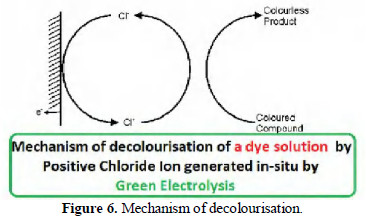
UV-visible absorption spectra
The UV-visible absorption spectra of dye solution before and after decolourisation are shown in Figs. 7, 8 and 9.
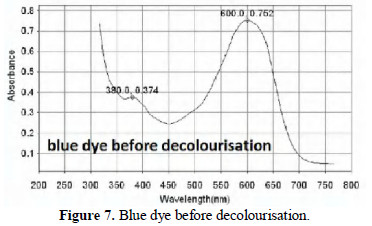
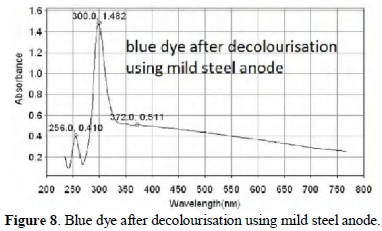
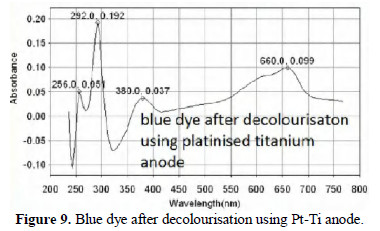
In Fig. 7 the absorption in the 300 nm region is due to π-π* electronic transition of the aromatic ring of the dyes. The peak at 600 nm is due to the chromophore group on the dye.
From Fig. 8 it is observed that when mild steel is used as anode, the chromophore has completely disappeared. The peak corresponding to aromatic rings is present. When platinised titanium is used as anode (Fig. 9), the aromatic rings and the chromophore are still present, but the intensity has decreased. The decolourisation efficiency is very high (99.8%). Based on the same principles, several dyes such as methyl orange [8], eriochrome black T [9], and orange 3 R dye [10] have been decolourised.
Synthesis of blue pigment (Prussian blue) from waste rusted mild steel Electrolysis
1 g of potassium ferrocyanide was dissolved in 10 mL of water in an undivided cell. 5 g of NaCl were added. A rusted mild steel was pickled and cleaned with water. It was used as anode. Graphite was used as cathode. A potential of 6 volts was applied for 5 minutes. Current density was 250 mA/cm2. The solution turned blue due to the formation of Prussian blue (Fig. 10).
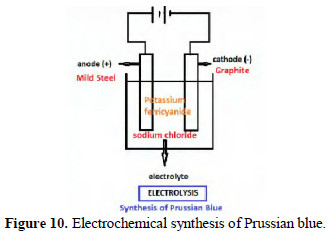
UV-visible absorption spectra
The UV-visible absorption spectrum of prussian blue (PB) prepared by chemical method (by mixing aqueous solutions of potassium ferricyanide and ferrous sulphate) is shown in Fig. 11.
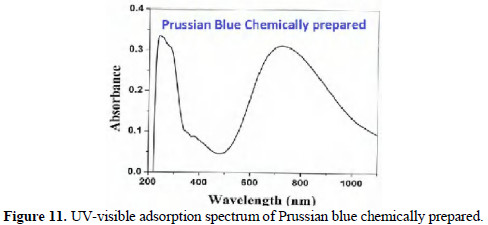
Peaks appeared at 275 nm and 750 nm.
It is observed (Fig. 12) that the UV-visible absorption spectrum of prepared electrochemically PB matches with that prepared chemically.
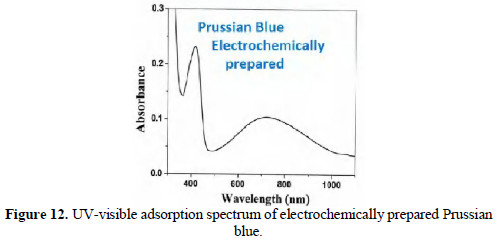
Thus, the formation of PB by electrochemical method from mild steel is confirmed.
Fluorescence spectra
The fluorescence spectra (λex =750 nm) of chemically and electrochemically prepared PB are shown in Figs. 13 and 14.
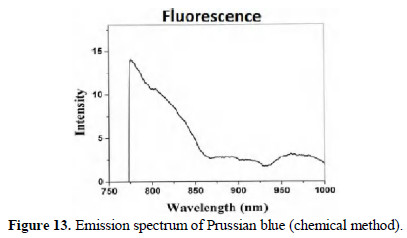
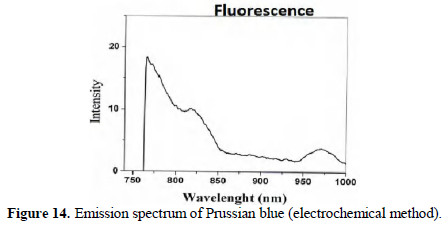
They closely resemble. Thus, formation of PB is confirmed.
FTIR spectra
The structures of Prussian blue are shown in Figs. 15 and 16.
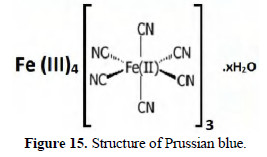
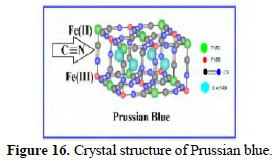
FTIR spectra of chemically and electrochemically prepared PB are shown in Figs. 17 and 18.
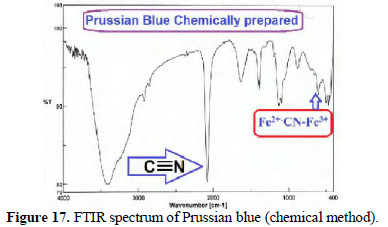
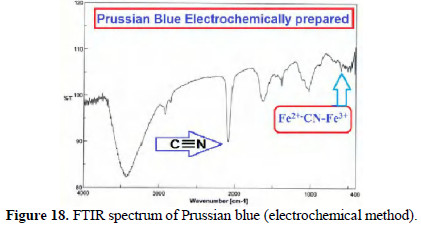
The presence of C ≡ N peak and Fe2+-CN-Fe3+ peak confirmed the formation of PB from mild steel.
SEM images
SEM images of chemically and electrochemically prepared PB are shown in Figs. 19 and 20.
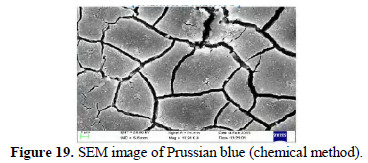
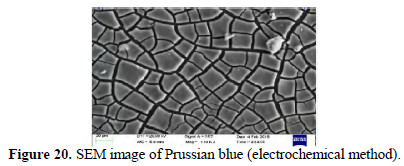
Squares and pentagons are seen. Some triangles are also seen.
Size of Prussian blue (PB) nano particles
The sizes of the PB nano particles prepared by chemical method are found to be 41.19 nm, 52.95 nm and 55.90 nm (Fig. 21).
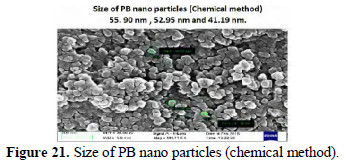
The sizes of the PB nano particles prepared by electrochemical method are found to be 41.12 nm, 52.86 nm, 58.74 nm and 64.61 nm (Fig. 22).
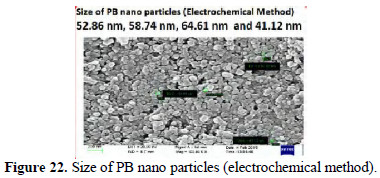
Squares and pentagons are seen. Some triangles are also seen.
Synthesis of silver nano particles
Silver nano particles have been prepared by electrolysis method (Fig. 23).
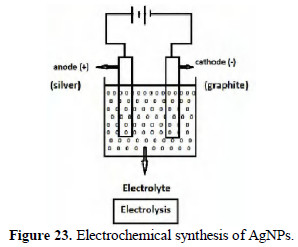
Pure silver rod was used as anode. Graphite was used as cathode. The electrolyte (reducing agent) was sodium potassium tartrate (SPT) and polyvinyl alcohol. When a potential of 6 volts was applied for 5 minutes (current density 50 mA/cm2), silver ions were released, which were reduced by SPT.
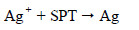
Pure The UV-visible absorption spectrum is shown in Figs. 24 and 25.
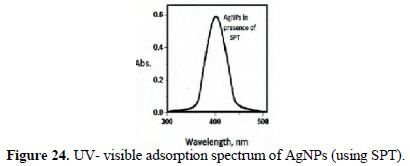
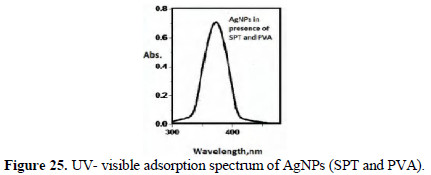
Fluorescence of AgNPs emission at 409.5 nm (violet-blue emission)
Peaks around 400 nm are due to AgNPs. They are found to be UV-fluorescence (λex = 400 nm) (Figs. 26 and 27).
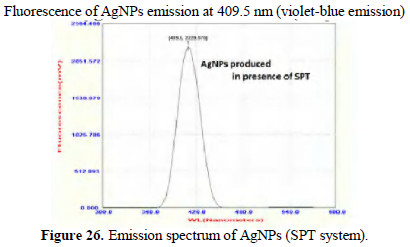
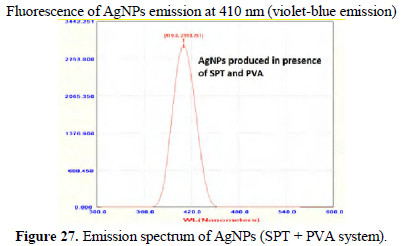
Fluorescence of AgNPs emission at 410 nm (violet-blue emission)
The sizes of AgNPs are found to be in the range of 75 to 100 nm (Fig. 28).
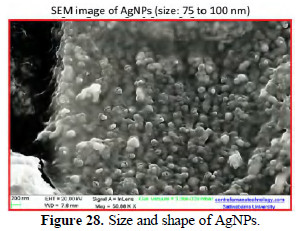
AgNPs are found to be spherical. Some flowers of AgNPs are also seen (Fig. 28).
EDAX spectrum
The EDAX spectrum confirms the presence of silver (Fig. 29).
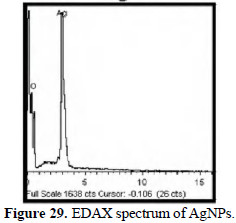
Formation of copper nanoparticles
In the electrolysis process, copper rod was used as anode. Graphite was used as cathode. The electrolyte was 1% of trisodium citrate (TSC) / sodium potassium tartrate (SPT). A potential difference of 6 volts was applied for 5 minutes. The current density was 50 mA/cm2.
The copper ions produced at anode were reduced by reducing agents such as SPT and TSC (Fig. 30).
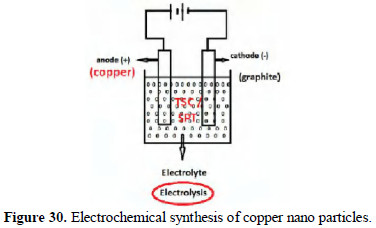
When TSC is used as reducing agent, the CuNPs show peaks at 228 nm, 275 nm and 681 nm in the UV-visible absorption spectrum (Fig. 31).
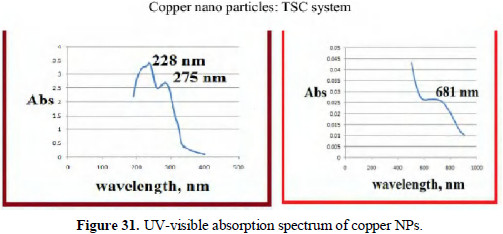
When SPT is used as reducing agent, peaks appear at 224 nm, 256 nm and 691 nm (Fig. 32).
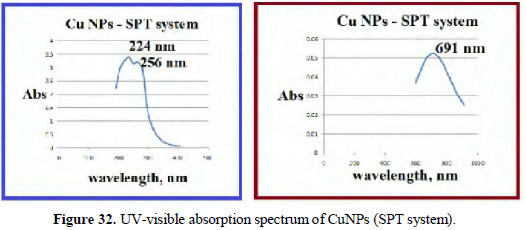
The slight shifts in the position of peaks may be due to the size of the nanoparticles. The CuNPs are found to be UV-fluorescent (λex) = 300 nm (Figs. 33 and 34).
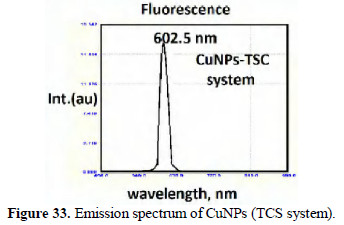
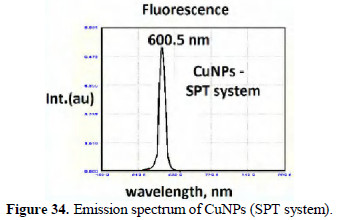
Electrochemical Hofmann rearrangement
Hofmann rearrangement involves conversion of acid amide into primary amine in the presence of hypohalite OCl-, OBr-(Cl2/NaOH, Br2/NaOH). In the present study benzamide was converted into a primary amine. 1 g of benzamide was taken as an undivided cell containing an aqueous solution of 5% NaCl solution. The anode was platinised titanium. The cathode was graphite (Fig. 35).
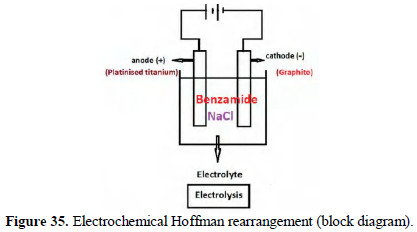
A potential difference of 6 volts was applied for 5 minutes. The current density was 50 mA/cm2. During electrolysis of NaCl, Cl2 and NaOH are produced. Thus, hypochlorite is produced. This has produced the Hofmann rearrangement climate. The resulting primary amine was diazotised and coupled with alkaline αnaphthol in NaOH solution, and β-napthol in NaOH solution. Dyes were produced. These dyes were matched with the dyes prepared from pure aniline.
The UV-visible adsorption spectra of the respective dyes were compared (Figs. 36 and 37; 38 and 39).
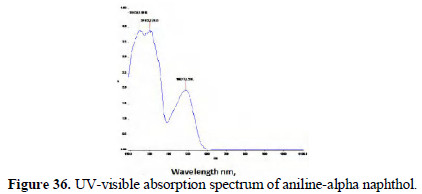
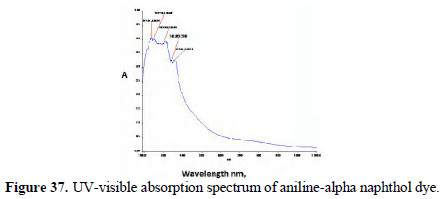
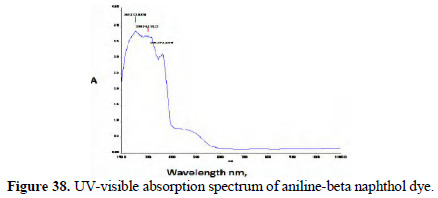
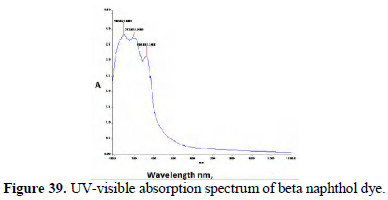
It is observed that, in the case of dyes obtained from pure aniline, a peak occurred around 475 nm range. But these peaks are absent or insignificant in the case of the spectra of dyes prepared by electrochemical method.
This may be due to the fact that the dyes prepared by electrochemical method are polymeric in nature (Figs. 40 and 41).
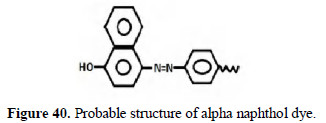
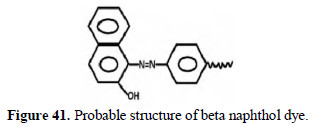
Further study in this line will lead to interesting conclusions. The dyes prepared from pure aniline and the dyes prepared by electrochemical method are found to be UV-fluorescent. (Figs. 42 and 43; 44 and 45).
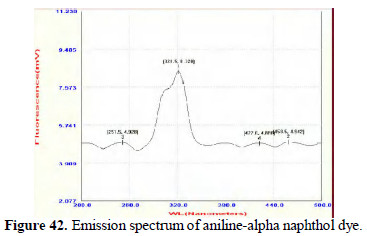
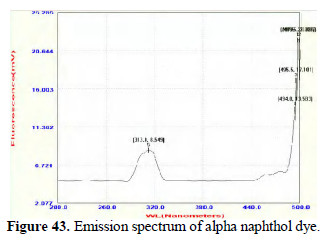
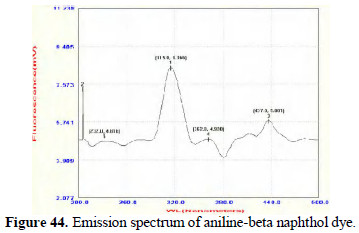
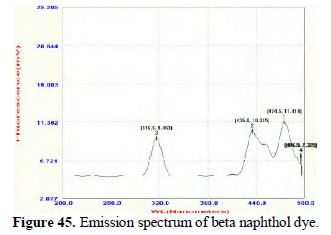
Electrochemical synthesis of poly aniline (PANI)
Aniline hydrochloride was dissolved in water and diluted to 100 mL (pH = 0.5). 5 g of NaCl were added. The solution was electrolysed (Fig. 46) using platinised titanium, and graphite cathode for a period of 5 minutes.
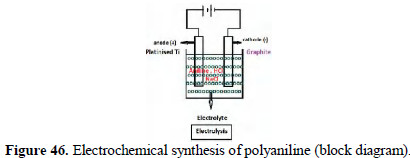
A potential difference of 6 volts was applied. The current density was 50 mA/cm2. Emerald green poly aniline was produced. In this method, conventional oxidizing agents were not used. The positive chloride ions produced in situ acted as oxidizing agent and initiated the polymerization process.
The UV-visible absorption spectrum of polyaniline produced showed peaks at 289 nm and 461 nm (Figs. 47 and 48).
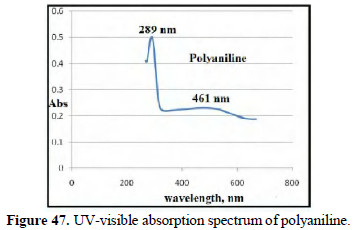
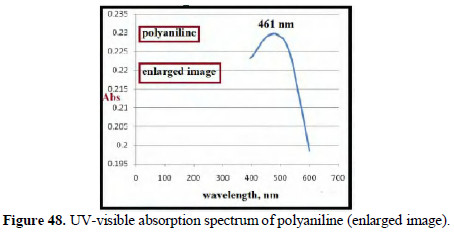
FTIR spectra of polyaniline The main poly aniline structure is shown in Fig. 49.
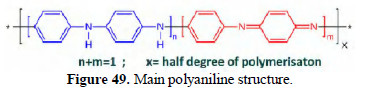
The FTIR spectra (KBr) of the poly aniline produced confirm the presence of benzenoid stretches (Fig. 50), C=N quinoidal units (Fig. 51) and -NH stretches (Fig. 52).
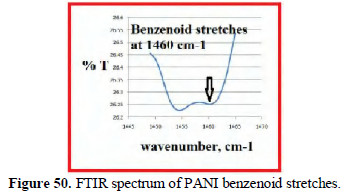
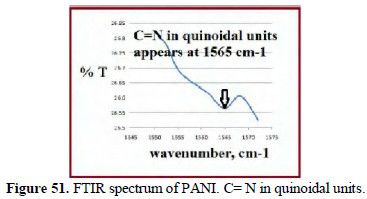
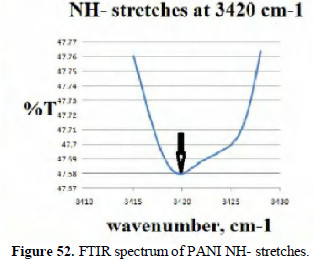
Thus, electrochemically generated poly aniline is confirmed.
Electrochemical green synthesis
By electrochemical method, dyes have been decolourised. Blue pigment has been produced. Silver and copper nano particles have been synthesized. Hofmann rearrangement has been effected. Poly aniline has been synthesized. All these processes use less hazardous chemicals. Safe chemicals and products are involved. Water is used as a solvent. There is no heating. Reaction time is only 5 minutes. Efficiency can be improved by increasing the voltage. Electric energy can be used again and again. Side products are not obtained. The Cl+ is used as catalyst and reagent. It is continuously generated. The products can be easily degraded. The experiments are free from pollution. The reactions can be started and stopped anytime.
All these facts support electro synthesis as a versatile green technique of the future.
References
1. Rajendran S, Trivedi DC. Synthesis Feb. 1995;153. [ Links ]
2. Chhabra M, Mishra S, Sreekrishnan TR. Biochem Eng J. 2015;93:17. [ Links ]
3. Pramila M, Manikandan S, Anju KS, et al. Sep Purif Technol. 2014;132:719. [ Links ]
4. Lin H, Zhang H, Hou L. J Hazardous Mater. 2014;276:182. [ Links ]
5. Joany RM, Shanthy P, Mary ACC, et al. Int J Nano Corr Sci Eng. 2015;2:16. [ Links ]
6. Howard JW, Derick CG. J Am Chem Soc. 1924;46:166. [ Links ]
7. Vivekanandan J, Ponnusamy V, Mahudeswaran A, et al. Arch Appl Sci Research. 2011;3:147. [ Links ]
8. Rajendran S, Devi MK, Regis APP, et al. Zastita Materijala. 2009;50:131. [ Links ]
9. Sathiyabama J, Rajendran S, Selvi JA, et al. Bull Electrochem. 2006;22:363. [ Links ]
10. Gowri S, Bama JS, Rajendran S, et al. Int J Nano Corr Sci Eng. 2014;1:2430. [ Links ]
Acknowledgements
The authors are thankful to their managements and Management of SRM Universty, Chennai, India, which is responsible for initiating this paper.
*Corresponding author. E-mail address: suasirajendran@gmail.com
Received July 15, 2016; accepted September 06, 2016