Serviços Personalizados
Journal
Artigo
Indicadores
-
Citado por SciELO
-
Acessos
Links relacionados
-
Similares em SciELO
Compartilhar
Portugaliae Electrochimica Acta
versão impressa ISSN 0872-1904
Port. Electrochim. Acta vol.35 no.6 Coimbra nov. 2017
https://doi.org/10.4152/pea.201706313
Ti/Pt/TiO2 electrodes prepared by DC Magnetron Sputtering: Environmental application on the degradation of the Acid Orange 7
Susana Serioa, Luis C. Silvaa , Maria E. M. Jorgeb, Silvio Ferreirac , Lurdes Ciriacoc,*, Maria J. Pachecoc and Ana Lopesc
a UNL - CEFITEC, Departamento de Física, Faculdade de Ciências e Tecnologia, Universidade Nova de Lisboa, 2829-516 Caparica, Portugal
b FCUL - CQB, Centro de Química e Bioquímica, Faculdade de Ciências, Universidade de Lisboa, 1749-016 Lisboa, Portugal
c UBI -FibEnTech-UBI, Departamento de Química, Universidade da Beira Interior, 6200-001 Covilhã, Portugal
Abstract
Titanium dioxide electrodes were prepared in three stages: (i) electrodeposition of Pt layer on a Ti substrate that was (ii) covered by a TiO2 thin film deposited by DC Magnetron Sputtering technique, at constant pressure of 0.8 Pa and oxygen partial pressure of 0.08 Pa, and (iii) annealed at 400 °C. The structural (XRD) characterization of the films was performed, showing a predominant anatase-TiO2 phase, where some peaks corresponding to the Ti/Pt substrate were also observed. The average crystallite size for the films was 44 nm, showing the nanocrystalline nature of these anatase-TiO2 films. The surface morphology of the films was studied by scanning electron microscopy and revealed agglomerates of nanosized grains or particulates, distributed over the substrate surface with a 'blooming flower-like' appearance. The nanosized grains result in an increase in active surface area and also promote the formation of porous TiO2 films. The performance of Ti/Pt/TiO2 as anode was evaluated in the electrodegradation of an azo dye, the acid orange 7 (AO7). Assays were run at 0.1, 0.25 and 1 mA cm-2, using AO7 concentration of 50 mg L-1, and after 12 h assays colour (484 nm) and chemical oxygen demand removals up to 97 and 70% were obtained, respectively. The energetic yield decreased with the increase in current density, which is typical from a process controlled by diffusion. The platinization of the substrate improves the adhesion of the TiO2 film, increasing its lifetime, and increases the conductivity of the films, decreasing the energetic costs of the process.
Keywords: TiO2 films; Acid Orange 7; Electrodegradation; DC Magnetron Sputtering; Decolourization.
1. Introduction
In order to search for efficient and low cost method to achieve water remediation, many studies have been developed, using different techniques. Among them, heterogeneous photocatalysis using suspensions of fine TiO2 particles have been performed successfully, in part due to the large surface area of the catalyst [1]. However, its application can be affected by difficulties in separating the fine oxide particles from the reaction medium. So, a better approach can be made by depositing the catalyst onto a substrate. If the substrate is conductor, TiO2 films, besides being used in photocatalytic studies, can also be utilized in electrochemical process, since anodic materials based on titanium oxides, as TiO2, are promising candidates due to their advantageous price, safety and toxicity, when compared to other possible anodic materials. The immobilisation of TiO2 Degussa P25 on glass has already been used as photoanode, with success, in the degradation of formic acid [2]. Degradation of paracetamol by different processes, such as photolysis, photocatalysis, electrocatalysis and photoelectrocatalysis, using modified reticulated vitreous carbon electrodes with TiO2, have been studied and the last process lead to a 95% degradation with 68% current efficiency, in 1 hour assay, 1.3 V vs. SCE and a Hg lamp with a 350 nm wavelength [3]. Studies performed with current and radiation have shown that the application of a positive potential bias to the TiO2 electrode improves the separation of photogenerated electrons and holes and also suppresses their recombination, leading to higher efficiency for pollutants degradation [4]. Zhang and co-workers have used TiO2 nanotube arrays electrode for the degradation of an azo dye, and concluded that photoelectrocatalytic process provided the most powerful way to degrade the methyl orange, achieving a 99.6% of dye removal after 90 min [5]. Liu and co-workers performed photoelectrochemical degradation of methyl orange by TiO2 nanopore arrays electrode and with TiO2 nanotube arrays electrode; comparing both electrodes, nanopore arrays electrode reveals enhanced photoelectrocatalytic activity for methyl orange degradation in comparison with nanotubes array electrode [6]. Zhang and co-workers propose a photoelectrocatalytic system for the degradation of methyl orange, using a TiO2 nanotube (TNT)/Ti electrode, which completely degrades 50 mg L-1 within 180 min with a mineralization efficiency of more than 80% [7].
Titanium is a commonly used substrate for metallic oxides electrodes. However, a passivation layer can be formed between substrate and the oxide during its application as anode. This layer determines the service-life of those electrodes. In order to overcome this problem and to significantly increase the useful life of the electrode, an inter-layer of another material is sometimes introduced. Platinum is a possible material that can prevent the passivation of the electrodes, increasing their service life-time [8].
In this work, Ti/Pt/TiO2 electrodes were prepared by three stages: electrodeposition of Pt on a Ti substrate and deposition of TiO2, by DC Magnetron Sputtering technique, followed by annealing. This technique allows the immobilisation of TiO2 in different kind of substrates, obtaining films with nanometric particles that present high uniformity. The TiO2 phase(s) obtained after quenching treatment depends on the preparation experimental condition, like oxygen partial pressure and type of substrate [9,10]. The performance of prepared Ti/Pt/TiO2 anodes was evaluated using as model compound an organic pollutant, the azo dye Acid Orange 7 (AO7).
2. Experimental details
2.1. Preparation and characterization of the Ti/Pt/TiO2 films
The TiO2 films used as electrodes in the electrodegradation assays were deposited by DC Reactive Magnetron Sputtering on platinized titanium substrates. The platinum coating was previously performed by electrodeposition [11]. For that, Ti substrates (99.7%, Sigma-Aldrich), with dimensions of 5 cm × 2.5 cm × 0.25 mm, were pre-treated with a mechanical polishing followed by etching with concentrated HCl in ebullition during 1 min. The substrates were then washed with double distilled water. Substrate platinization was performed in a one compartment double wall cell, connected to a thermostatic water-bath, which enabled the recirculation of water at 65 °C. The cell contained a chloroplatinic acid (99.9%, Sigma-Aldrich) solution and a current density of 250 mA cm-2 was applied [11], using the Ti substrate, as cathode, between two platinum plates, as anodes.
For the production of the TiO2 films on Ti/Pt substrate, a titanium disc (99.99% purity) having 64.5 mm of diameter and 4 mm of thickness was used as sputtering target. A turbomolecular pump was used to achieve a base pressure of 10-5 Pa (before introducing the gas mixture). The films were reactively sputtered in a mixture of 99.99% pure argon and 99.99% pure oxygen, and the partial pressures of these gases were separately controlled by mass flow controllers. The total pressure, PT, during the deposition was kept constant at 0.8 Pa and the partial pressure of oxygen was set at 0.08 Pa (i.e. 10% of PT). The sputtering power was kept at 1000 W and the deposition time was 80 min. Before the sputter-deposition step of the films, a movable shutter was interposed between the target and the substrates, and the target was pre-sputtered in Ar atmosphere for 5 min to clean the target surface. The target-to-substrate distance was kept constant at 100 mm. To improve the effective area the TiO2 films were deposited in both sides of the platinized titanium substrates. In order to allow crystalline growth the as-sputtered films were annealed in air at 400 °C for 4 h in a tubular furnace, in agreement with previous studies [9,12].
The structural characterization of the films was carried out by X-ray diffraction (XRD) on a Philips Analytical PW 3050/60 X'Pert PRO (theta/2 theta) equipped with X'Celerator detector operating with monochromatized CuKα radiation in Bragg- Brentano geometry. Diffractograms were obtained by continuous scanning in a 2q-range of 20° to 90° with a 2q-step size of 0.02° and a scan step time of 20 s. The average crystallite size was calculated from the broadening of (101) diffraction peak using the Scherrer's formula, D = 0.9λ/B cos θ, where λ is the wavelength of Cu Kα radiation, B is the full width at half maximum (FWHM) of XRD peaks and θ the Bragg diffraction angle of the peak (101). Correction for the line broadening by the instrument was applied using silicon as a reference and the relationship B2 = BM2 - BS2, where BM and BS are the measured widths, at half maximum intensity, of the lines from the sample and the reference, respectively [13].
Voltammetric studies and electrocatalytic assays were performed with a potenciostat/galvanostat VoltaLab PGZ 301, in a one-compartment cell with three electrodes: a working electrode, Ti/Pt/TiO2, with an immersed geometric area of 2 cm2, an auxiliary electrode that was a platinum plate with 1 cm2 of geometric area each side, and a reference electrode, Ag/AgCl, KClsat. For the electrocatalytic assays, the anode was a Ti/Pt/TiO2 and the cathode was a stainless steel foil, both with a geometric area of 20 cm2. All the degradation assays were performed at room temperature, with stirring, being the processed solution volume 200 mL and the applied current densities 0.1, 0.25 and 1.0 mA cm-2. The AO7 aqueous solutions had an initial dye concentration of 50 mg L-1, and contained Na2SO4 (5 g L-1) as background electrolyte. The reagents AO7, C16H11N2NaO4S (Aldrich, >85%) and Na2SO4 (Merck, 99.5%) were used as purchased. The AO7 degradation assays were periodically sampled and monitored by UV-Vis absorption spectrophotometry, in the range 200-600 nm, using a Shimatzu UV-1800 spectrophotometer. Chemical oxygen demand (COD) determinations, using closed reflux and titrimetric method, were also performed, according to standard procedures [14].
Prior to the degradation assays, the AO7 solutions where left for one hour, with stirring, in the electrochemical cell completely assembled. UV-Vis spectra of the initial solutions and of the samples collected after one hour stirring were compared, in order to exclude removal of the dye from solution due to adsorption onto the anodes' surface.
3. Results and discussion
3.1. Structural properties
Since the as-deposited films XRD analysis revealed amorphous nature, the films were annealed at 400 °C during 4 h to allow anatase TiO2 crystallization. Similar behaviour was previously described for the TiO2 films deposited by DC reactive magnetron sputtering but on glass substrates [9,12].
Figure 1 shows the XRD pattern of the annealed TiO2 films deposited on the platinized titanium substrate. The observed peak at 2q = 25.3° corresponds to the (101) plane of the anatase-TiO2 phase.
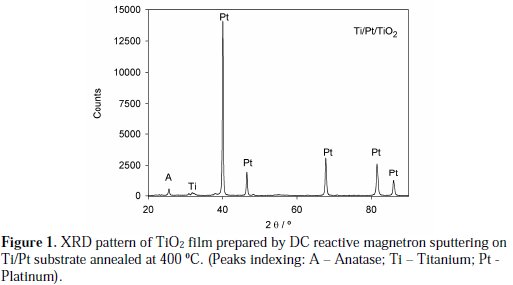
Peaks corresponding to the Ti/Pt substrate can also be detected. The average crystallite sizes for the films were calculated from the peak width at half peak height of the diffraction peak (101), based on the instrumental broadening correction. The obtained value for the average crystallite size was 44 nm, showing the nanocrystalline nature of these anatase TiO2 films.
3.2. Morphological properties
Figure 2 shows SEM images of the TiO2 films deposited over the Pt/Ti substrates by DC Reactive Magnetron Sputtering and annealed at 400 °C.
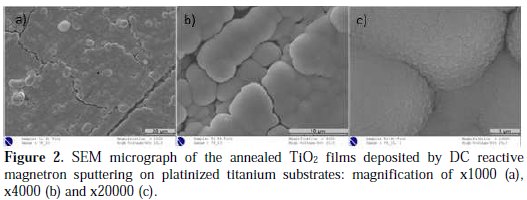
The surface morphology of the films consists of agglomerates of grains or particulates distributed over the substrate surface with a 'blooming flower-like' appearance. A more detailed observation of this image evidences the formation of the nanosized grains protruding on the submicrometer-sized grains. The nanosized grains result in an increase in active surface area and also promote the formation of porous TiO2 films, which is a key parameter in the electrodegradation studies.
3.3. Voltammetric study
Figure 3 presents the voltammograms of two different working electrodes, Ti/Pt and Ti/Pt/TiO2, in an aqueous solution of Na2SO4 5 g L-1, acquired at a scan rate of 20 mV/s.
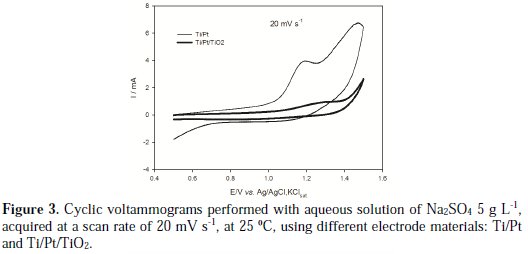
It can be observed that the platinized titanium presents higher current intensity than the platinized titanium substrate covered with the TiO2 film. This is due to the higher conductivity of the platinum film. An anodic peak is observed at 1.2 V vs Ag/AgCl,KClsat, which can be attributed to one of the steps of the platinum oxidation: the adsorption of oxygen followed by the formation of platinum oxide [15]. In the Ti/Pt/TiO2 electrode these anodic peaks are also present, since the TiO2 film is thin and porous, allowing the contact between the solution and the inner Pt layer that possesses high adsorptive characteristics. However, the deposition of the TiO2 layer reduces the conductivity of the electrode and also the ability of the oxygen to be adsorbed on the electrode's surface, thus reducing the current intensity for the adsorption process at the Ti/Pt/TiO2 electrode. On the other hand, there is an increase in the potential for oxygen evolution when the platinized substrate is covered with the TiO2 film, which may improve the ability of the material to be used in environmental applications, because there is an increase in current efficiency towards pollutants electrodegradation when lower tendency for oxygen evolution is observed.
3.4. Electrodegradation assays
Figure 4 presents the UV-Vis spectra for the samples collected during the AO7 degradation assay performed with the Ti/Pt/TiO2 anode at an applied current density of 1 mA cm-2.
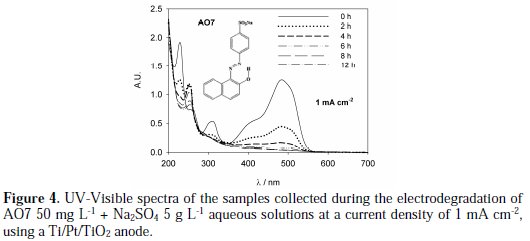
It can be observed a regular decay in absorbance in the visible region due to the broken of the azo bond, which leads to an increase in the absorbance at 250 nm, especially at 2 h assay, due to the consequent increase in the concentration of aromatic byproducts [16]. Regarding the influence of the current density on the AO7 degradation rate, it can be observed that an increase in the current density leads to an increase in the absorbance removal rate, at 229 and 484 nm, as well as in the COD removal rate (Figure 5 and Table 1).
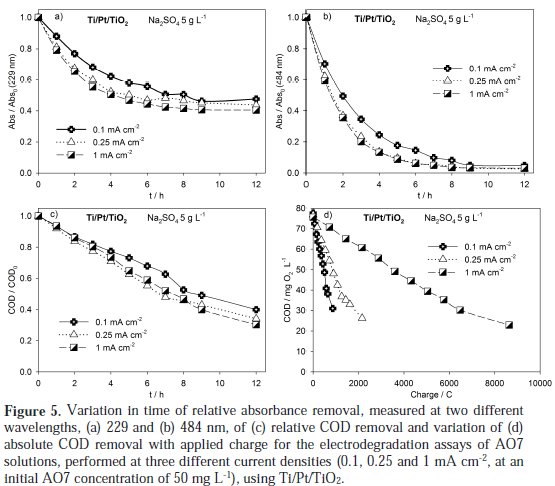
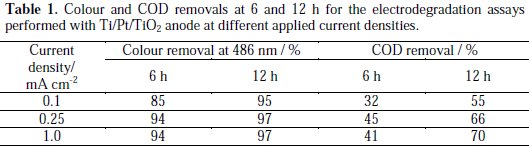
However, when COD variation during the assays is plotted against electrical charge, it can be concluded that in the assay run at 0.1 mA cm-2 the current efficiency is much higher. This is typical of processes controlled by diffusion, where the increase in current density corresponds to a decrease of the current efficiency due to the formation of oxygen from water. The small increase in the degradation rate with current density is due to an increased formation of hydroxyl radicals that help in the AO7 mineralization.
In a previous work, Ti/TiO2 films were prepared also by DC Magnetron Sputtering Technique, with 15% partial pressure of O2, and the predominant phase was anatase, although rutile phase was also found [10]. Other previous study [12] refers that the preparation of TiO2 films on glass by the same technique, using a partial pressure of 10%, favour the anatase phase, which presents higher catalytic effect. The TiO2 films prepared in this work supported on platinized titanium, using a partial pressure of O2 of 10%, led to anatase phase, without any evidence of rutile presence, which improves the catalytic effect of the film.
By comparing the electrodegradation results obtained in this work with those obtained with a Ti/TiO2 anode prepared by the same technique [10], after 6 h assay it can be observed that, in the assays performed with Ti/Pt/TiO2 anode, the absorbance removal at 484 and 229 nm is enhanced relatively to the assays performed with Ti/TiO2 anode, for both current densities utilized. However, the effect of the anode material in the COD removal is not so clear, probably due to different mechanisms of the AO7 degradation for different current densities. Platinum presents high adsorptive characteristics that can reduce the degradation rate; even covered with titanium oxide, the porosity of the film can allow higher adsorption of the formed metabolites. On the other hand, platinization increases the conductivity of the films and improves the adhesion of titanium dioxide on the substrate, improving the mechanical stability of the TiO2 and allowing the application of higher current densities without destroying TiO2 films.
Conclusions
Ti/Pt/TiO2 anodes were obtained successfully by depositing TiO2 on Ti/Pt substrates, by DC Magnetron Sputtering Technique, followed by annealing. At the experimental conditions utilized during the TiO2 deposition over the platinized Ti substrate, only the anatase phase was observed, with an average crystallite size of 44 nm. Cyclic voltammetry studies have shown anodic peaks in sodium sulphate solutions at both Ti/Pt and Ti/Pt/TiO2 materials. These peaks, attributed to the adsorption of oxygen followed by the formation of platinum oxide, present lower current intensities for equal applied potential difference at Ti/Pt/TiO2 anode, since Pt is covered by a porous TiO2 layer. On the other hand, the deposition of a TiO2 layer over the platinized titanium increases the potential for oxygen evolution.
Regarding the environmental application of TiO2 on the electrodegradation of AO7 solutions, it was observed that colour removal at 484 nm is almost total at the end of assays, meaning that azo bond (-N=N-) is easily broken. Absorbance removal at 229 nm is lower, since benzenic and naftalenic rings are not so easily opened. Also, the increase in current density from 0.1 to 1 mA cm-2 do not significantly increases absorbance or COD removal rates, but the specific electric charge consumption increases with current density, meaning that, at the experimental conditions utilized in this study, the degradation process is diffusion controlled.
References
1. Chatterjee D, Dasgupta S. J Photochem Photobiol C-Photochem Rev. 2005;6:186. [ Links ]
2. Byrne JA, Davidson A, Dunlop PSM, et al. J Photochem Photobiol A-Chem. 2002;148:365. [ Links ]
3. Valdez HCA, Jimenez GG, Granados SG, et al, Chemosphere. 2012;89:1195. [ Links ]
4. Oliveira HG, Nery DC, Longo C. Appl Catal B-Environ. 2010;93:205. [ Links ]
5. Haizhang Z, Yuan Y, Shi G, et al. Environ. Sci. Technol. 2007;41:6259. [ Links ]
6. Liu Y, Gan X, Zhou B, et al. Water Sci. Technol. 2010;62:2783. [ Links ]
7. Zhang A, Zhou M, Liu L, et al., Electrochim Acta.2010;55:5091. [ Links ]
8. Santos D, Pacheco, Gomes A, et al. J Appl Electrochem. 2013;43:407.
9. Serio S, Jorge ME, Coutinho ML, et al. Chem Phys Lett 2011;508:71. [ Links ]
10. Serio S, Silva LC, Jorge ME. Environ Eng Manage J. (in press). [ Links ]
11. Andrade LS, Rutuolo LAM, Rocha-Filho RC, et al. Chemosphere. 2007;66:2035. [ Links ]
12. S. Serio S, Jorge ME, Maneira MJP, et al. Chem Phys. 2011;126:73.
13. Cullity BD, Elements of X-ray Diffraction, 2nd edition, Addison-Wesley, Reading, MA 1978. [ Links ]
14. Eaton A, Clesceri L, Greenberg A. Standard methods for examination of water and wastewater, 21st ed., APHA, AWWA, WEF, Washington, 2005. [ Links ]
15. Jerkiewicz G, Vatankhah G, Lessard J., et al. Electrochem Acta. 2004;49:1451. [ Links ]
16. Carvalho C, Fernandes A, Lopes A. et al. Chemosphere, 2007;67:1316. [ Links ]
Acknowledgements
The authors acknowledge the financial support from FEDER, Programa Operacional Factores de Competitividade - COMPETE, and FCT, for the projects PTDC/AACAMB/ 103112/2008, PEst-OE/CTM/UI0195/2011, UID/FIS/00068/2013 and PEstOE/ QUI/UI0536/2011. S. Serio acknowledges the financial support from FCT for the Programme Ciencia 2007.
*Corresponding author. E-mail address: lciriaco@ubi.pt
Received December 21, 2016; accepted September 13, 2017