Serviços Personalizados
Journal
Artigo
Indicadores
-
Citado por SciELO
-
Acessos
Links relacionados
-
Similares em SciELO
Compartilhar
Portugaliae Electrochimica Acta
versão impressa ISSN 0872-1904
Port. Electrochim. Acta vol.36 no.2 Coimbra mar. 2018
https://doi.org/10.4152/pea.201802077
The Inhibition Action of Essential Oil of J. Juniperus Phoenicea on the Corrosion of Mild Steel in Acidic Media
Y. Elkhotfia,* , I. Forsala , E.M. Rakiba and B. Mernarib
a Organic and Analytical Chemistry Laboratory, University Sultan Moulay Slimane, Faculty of Sciences and Technologies, Beni Mellal, Morocco
b Coordination and Analytical Chemistry Laboratory, Chouaib Doukkali University, Faculty of Sciences El Jadida, Morocco
Abstract
Essential oil of Juniperus phoenicea (Cupressaceae) is extracted from the natural plant collected in Morocco. Extracted by distillation, its inhibiting action on the corrosion of mild steel in 1 M acidic media has been investigated by weight loss and various electrochemical techniques. Obtained results reveal that this naturally occurring substance is a very good inhibitor. The inhibition efficiency was found to increase with the oil content, attaining 83% at 1500 ppm. The oil of Juniperus phoenicea acts as a mixed-type inhibitor. The adsorption isotherm and the thermodynamic data of adsorption and activation are herein determined and discussed.
Keywords: Steel, acid solution, weight loss, acid corrosion, adsorption.
Introduction
Environmental technology, also called green or clean technology, is the application of environmental science to conserve the natural environment and resources, and to curb the negative impacts of human involvement. Sustainable development is the core of environmental technologies. Owing to increasing ecological awareness, strict environmental regulations and the need of environmentally friendly processes, research is now focused on the development of substitute nontoxic biodegradable natural compounds [1].
In this frame, most of the naturally occurring substances are safe and can be extracted by simple and cheap procedures. Recent literature is full of researches which test different extracts for corrosion inhibition applications [2-12]. Many oils extracted from different parts of different plants are rich in aroma compounds. Many of these naturally occurring substances proved their ability to act as inhibitors for the corrosion of different metals and alloys in different aggressive media. To value the inhibitory performance of the essential oil of Juniperus phoenicea, weight loss and electrochemical techniques (AC impedance and polarization techniques) have been employed. The effect of concentration and temperature on the inhibition efficiency has been examined. The thermodynamic parameters for both dissolution and adsorption processes were calculated and discussed.
Materials and methods
Natural oil of Juniperus phoenicea
The essential oil of Juniperus phoenicea, endemic to Morocco, was isolated by distillation, and analyzed by gas chromatography-mass spectrometry (GC-MS) [13].
Specimens
Mild steel specimens containing 0.09% P, 0.38 % Si, 0.01 Al, 0.05 % Mn, 0.21% C, 0.05% S and the remainder Fe, were used for gravimetric and electrochemical measurements. These steel specimens were mechanically cut into 1 cm × 5 cm × 0.06 cm dimensions for weight loss and electrochemical experiments. Prior to all measurements, they were mechanically polished on wet SiC paper (grade 400 - 800 - 1200), rinsed with double distilled water, ultrasonically degreased in ethanol for 5 min, and dried at room temperature.
Electrolytes
Aggressive solutions of 1 M HCl were prepared by dilution of analytical grade 37% with double distilled water. The concentration range of the employed inhibitors has varied from 800 ppm to 1500 ppm in 1 M HCl, and the used electrolyte was 50 mL.
Methods
Gravimetric measurement
The weight loss of steel, with and without the addition of different oil concentrations, was determined after immersion in acid over 24 h at 30 °C, and the percentage inhibition efficiency (η%) was calculated from:

where w0 and w are the values of the corrosion weight loss of steel after immersion in solutions, respectively, without and with inhibitor.
Electrochemical measurement
Electrochemical experiments were conducted using impedance equipment (Tacussel-Voltalab PGZ 100), and controlled with Tacussel corrosion analysis software model Voltamaster 4.
A conventional three electrode glass cell assembly with a mild steel rod was used as working electrode (we); a platinum foil of 3-3 cm as auxiliary electrode; and (CE) Hg/Hg2Cl2/KCl saturated (SCE) for the 1 M HCl medium, were used as reference electrodes. AC impedance measurements were carried-out at Ecorr after immersion in a solution without bubbling, and the rectangle surface of the steel, with 1 cm2 exposed to the solution, was used as working electrode. After the determination of steady state current at a given potential, peak to peak sine wave voltage (10 mV), at frequencies between 100 KHz and 10 mHz, was superimposed on the open circuit potential. Computer programs automatically controlled the measurements performed at rest potentials after 30 min of exposure. The impedance diagrams are given in the Nyquist representation. Values of Rtc and Cdl were obtained from Nyquist plots. For polarization curves, potentiodynamic polarisation studies were performed with a scan rate of 1 mV.s-1 in the potential range from -750 mV to -100 mV, relative to the corrosion potential.
Results and discussion
Weight loss measurements
The inhibitor efficiency increased with the oil content. The inhibition was estimated to be superior to 78 % in 1 M HCl, even at different concentrations, and the optimum concentration for maximum efficiency was found to be 1500 ppm of oil.
The inhibition efficiencies calculated from the weight loss measurements for different amounts of essential oil of Juniperus phoenicea in 1 M HCl are summarized in Table 1.
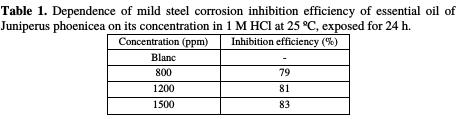
Polarization measurements
The polarization curves of steel in 1 M HCl, in the absence and presence of the essential oil at 25 °C, are presented in Fig. 1.
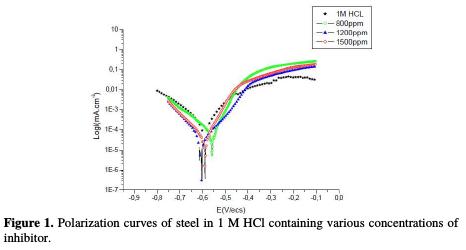
The collected parameters deduced from the polarization curves, such as corrosion potential (Ecorr), corrosion current (icorr), Tafel slopes (bc, ba), and percentage inhibition efficiency, are shown in Table 2.
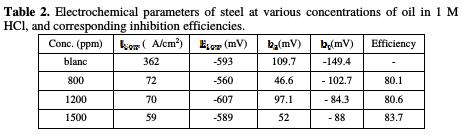
Examination of Fig. 1 and Table 2 shows that. The cathodic current density decreases with the natural substance concentration. The slopes of cathodic Tafel lines, and the corrosion potential remain almost constant upon the addition of the inhibitor concentration. This result indicates that the reduction mechanism of the hydrogen ion is modified in the oil's presence [14-15]. In the anodic range, the polarization curves in steel in 1 M HCl, with and without oil, show that the inhibitor presence decreases the current density. This fact means that the essential oil of Juniperus phoenicea inhibits anodic reaction and acts a mixed- type inhibitor.
The inhibition efficiency increases with the inhibitor concentration, attaining 83% at 1500 ppm of the natural substance.
Electrochemical impedance spectroscopy (EIS)
The corrosion of mild steel in an acidic solution, in the presence of a natural substance, was investigated by EIS. Nyquist plots of steel, in inhibited and uninhibited acidic solutions containing various concentrations of oil, are shown in Fig. 2.
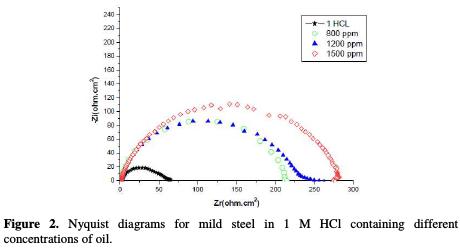
The obtained impedance diagrams are not perfect semicircles, and this difference has been attributed to frequency dispersion [16-18].
The transfer resistance values (Rtc) are calculated from the difference in impedance at lower and higher frequencies [19]. To obtain the double layer capacitance (Cdl), the frequency at wich the imaginary component of the impedance (-Zmax) is found, and Cdl values, are obtained from the equation:

The inhibition efficiency is calculated by transfer resistance, as follows:
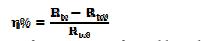
where Rtc and Rtc0 are the transfer resistances for mild steel in 1 M HCl, respectively, with and without inhibitor.
It is found (Table 3) that, as the oil concentration increases, the Rtc values increase (Fig. 3), but the Cdl values tend to decrease (Fig. 4).
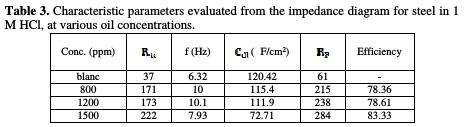
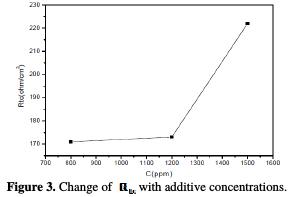

The decrease in values is due to the adsorption of aroma compounds onto the metal surface [20]. The electrochemical study confirms the results of the weight loss measurements. The Juniperus phoenicea's oil appears to be a good inhibitor in 1 M HCl, with a maximum efficiency of 83%.
The effect of temperature on the anti-corrosion effectiveness of oil, studied at various concentrations in the temperature domain of 298-333 K, at 2 h of immersion, is summarized in Table 4.
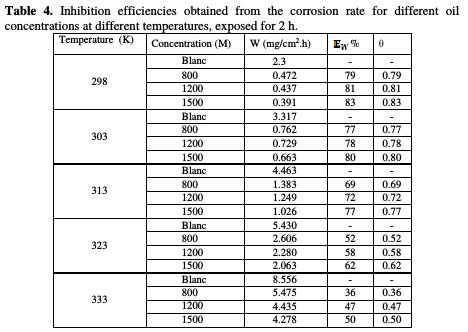
The collected curves in Fig. 5 show the evolution of the corrosion rate (W) with oil concentration (C), at different temperatures.
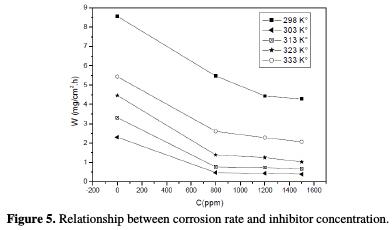
Fig. 5 indicates that, at a given oil concentration, the corrosion rate of steel increased with temperature. The increase is more pronounced at weak concentrations. The results also indicate that, for a given temperature, the corrosion rate of steel decreased with increasing inhibitor concentration. The values of inhibition efficiency obtained from weight loss for different inhibitor concentrations, and at various temperatures, in 1 M HCl, are given in Table 4 and Fig. 5. The results show that the inhibition efficiency decreases with increasing temperature, indicating that the dissolution of steel at higher temperatures predominates on the adsorption of aroma compounds onto the surface.
The activation kinetic parameters, such as energy (Ea), enthalpy (ΔHa0) and entropy (ΔSa0), may be evaluated from the effect of temperature on the inhibitor, using Arrhenius law (Eq. 4) and the alternative formulation of Arrhenius equation (Eq. 5) [21].


where A is the Arrhenius pre-exponential factor, T the absolute temperature, Ea the activation corrosion energy for the corrosion process, h the Planck's constant, N the Avogadro's number, ΔSa0 the entropy of activation, ΔHa0 the enthalpy of activation, and I is the corrosion rate of steel.
The apparent activation energies (Ea) and pre-exponential factors (A) are calculated by linear regression between ln(I) and 1/T (Fig. 6).
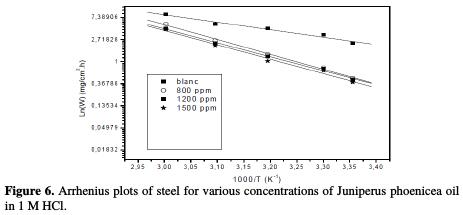
The apparent activation corrosion energies in the absence and presence of 1500 ppm of Juniperus phoenicea oil were found to be, respectively, 28.17 and 52.52 kJ/mol. The pre-exponential factors (A) and Ea show the same trend. The increase in Ea, in the oil presence, may be interpreted as the likely specific interaction between the iron surface and aroma compounds [22], that occurs in the first stage [23]. The increase in activation energy can be attributed to an appreciable decrease in the adsorption of the aroma compounds onto the mild steel surface with an increase in temperature. A corresponding increase in the corrosion rate occurs because of the greater area of metal that is consequently exposed to the acid environment.
Fig. 7 shows plots of ln(w/T) against 1/T.
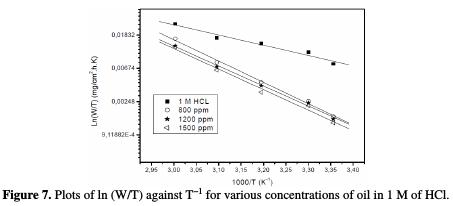
Straight lines are obtained with a slope of (-ΔHa0/R) and an intercept of (ln R/Nh + ΔSa0/R), from which the values of ΔHa0 and ΔSa0 are calculated (Table 5).
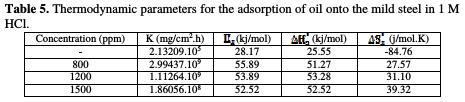
The obtained values of ΔHa0 are 25.55, 51.27, 53.28 and 52.52 Kj/mol for free acid and 800, 1200 and 1500 ppm in acid added with oil, respectively. The positive signs of the enthalpies, ΔHa0, reflect the endothermic nature of the steel dissolution process, and mean that the dissolution of steel is difficult [24].
The positive values of entropies (ΔSa0) in the inhibitor presence imply that the activated complex in the rate determining step represents an association rather than a dissociation step, meaning that an increase in disordering takes place on going from the reactants to the activated complex [25].
Adsorption isotherm
The surface coverage, θ, was calculated according to the following equation:

Surface coverage values (θ) for the inhibitor were obtained from the weight loss measurements for various concentrations at different temperatures (303-333 K), as shown in Table 4.
The dependence of the fraction of the covered surface, θ, obtained by the ratio Ew/100, on the concentration (C) (Fig. 8), was graphically fitted for Langmuir, Temkin, Frumkin and Freundlich adsorption isotherms.
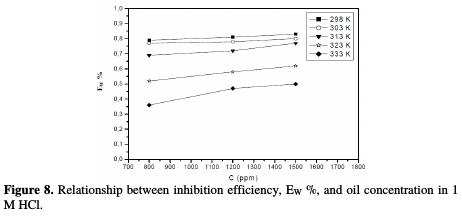
The models considered were [26].




where Kads is the equilibrium constant of the adsorption process, C the inhibitor concentration and f the factor of energetic inhomogeneity [27].
The modes of adsorption mainly depend on the chemical structure of the active molecules of this oil. The adsorption mainly depends on the electronic and structural properties of aroma compounds, such as functional groups, steric factors, aromaticity, and electron density [28-29].
Conclusion
1. The essential oil of Juniperus phoenicea acts as a good inhibitor for the corrosion of steel in acidic media.
2. Essential oil of Juniperus phoenicea acts as a mixed-type inhibitor.
3. The inhibition efficiency of oil decreases with the rise in temperature within the range 298-333 K.
4. The results obtained from weight loss, potentiodynamic polarization and impedance spectroscopy are in good agreement.
References
1. Oguzie EE, Li Y, Wang FH. J Colloid Interface Sci. 2007;310:90. [ Links ]
2. El-Etre AY, Abdallah M, El-Tantawy ZE. Corros Sci. 2005;47:385. [ Links ]
3. El-Etre AY. Corros Sci. 2003;45:2485. [ Links ]
4. Avwiri GO, Igho FO. Mater Let. 2001;57:3705. [ Links ]
5. El-Etre AY, Abdallah M. Corros Sci. 2000;42:731. [ Links ]
6. Martinez S, Stern I. Appl Surf Sci. 2002;199:83. [ Links ]
7. El-Etre AY, Abdallah M. Corros Sci. 2000;42:731. [ Links ]
8. Chetouani A, Hammouti B. Bull Electrochem. 2003;19:23. [ Links ]
9. Chetouani A, Hammouti B. Bull Electrochem. 2004;20. [ Links ]
10. Benabdellah M, Benkaddour M, Hammouti B, et al. 2006;52:6212.
11. Znini M, Bouklah M, Majidi L, et al. Int J Electrochem Sci. 2011;6:691. [ Links ]
12. Chaieb EL, Bouyanzer A, Hammouti B, et al. Appl Surf Sci. 2005;249:183. [ Links ]
13. Achak N, Romane A, Abbad A, et al. J Ess Oi Bear Plan. 2008;11:137. [ Links ]
14. Lagreneé M, Mernari B, Chaibi N, et al. Corros Sci. 2001;43:951.
15. Bentiss F, Traisnel M, Vezin H, et al. Corros Sci. 2003;45:371. [ Links ]
16. Mansfeld F, Kending MW, Tsai S. Corrosion. 1981;37:301. [ Links ]
17. Hsu CH, Mansfeld F. Corrosion. 2001;57:747. [ Links ]
18. Bentiss F, Bouanis M, Mernari B, et al. Corrosion. 2007;273:3696. [ Links ]
19. Tsuru T, Haruyama S, Gijutsu B. J Japan Soc Corros Eng. 1978;27:573. [ Links ]
20. Bentiss F, Lebrini M, Lagrenée M. Corros Sci. 2005;47:2915.
21. Arab ST, Noor EA. Corrosion. 1993;49:122. [ Links ]
22. Popova A, Sokolova E, Raicheva S, et al. Corros Sci. 2003;45:33. [ Links ]
23. El Sherbini EF. Mater Chem Phys. 1999;60:286. [ Links ]
24. Gomma MK, Wahdan MH. Mater Chem Phys. 1995;39:209. [ Links ]
25. M. Elachouri M, Hajji MS, Salem M, et al. Corrosion. 1996;52:103.
26. Bouklah M, Hammouti B, Lagrenée M, et al. Corros Sci. 2006;48: 2831. [ Links ]
27. Popova A, Christov M, Raicheva S, et al. Corros Sci. 2004;46:1333. [ Links ]
28. Bockris JO'M, Young B. J Electrochem Soc. 1991;138:2237. [ Links ]
29. Touhami F, Aouniti A, Kertit S, et al. Corros Sci. 2000;42:929. [ Links ]
Acknowledgements
The authors are pleased to acknowledge Laboratory Electrochemistry and Corrosion Studies, Faculty of Science Kenitra, for technical assistance.
*Corresponding author. E-mail address: elkhotfi@gmail.com
Received December 28, 2016; accepted July 1, 2017