Introduction
Metal corrosion is a major industrial problem that has received a considerable amount of attention. The prevention of corrosion plays an important role in economics and safety. The use of inhibitors is one of the most practical methods to protect metals against corrosion, especially in acidic media1. Among these inhibitors, N-heterocyclic compounds are considered to be the most effective corrosion inhibitors. Up to now, many N-heterocyclic compounds with one or several N heteroatoms have been reported as good corrosion inhibitors for iron or steel in acidic media2, such as imidazoline derivatives3-5, pyridine derivatives6-8, pyrimidine derivatives9, benzimidazole derivatives10-14 and quinoline derivatives15.
Pyridinylbenzoxazol compound shows two anchoring sites suitable for surface bonding: the nitrogen atom with sp2 electron pair and the aromatic rings16. It is a general assumption that the adsorption of the organic inhibitors at the metal/surface interface is the first step in the mechanism of the inhibitor action. This adsorption produces a uniform film on the metal surface, which reduces or prevents contact with the corrosive medium17.
The present paper reports the study of the inhibitive action of the 2-(pyridin-2-yl)benzoxazol (L1), 2-(benzoxazol-2-yl)quinoline (L2) and2-(5-(trifluoromethyl)pyridin-2-yl) benzoxazol (L3) on the corrosion of steel in molar hydrochloric acid. The steel corrosion behaviour has been evaluated by different techniques: weight loss, potentiodynamic polarisation, EIS measurements and theoretical calculations. The molecular structures of these compounds are presented in Fig. 1.
Material and methods
Synthesis of Benzoxazol
The synthesis of these polyhetero-aromatics compounds using inexpensive and environementaly attractive methods (step and atom economy, limited quantity of toxic substances) is one of the major challenges in organic chemistry.
In this context, the Pyridinylbenzoxazol used in this study was synthetized using direct C-H bond activation of benzoxazol catalyzed by palladium, using halo-aryl halides as coupling partner. This method appeared as a greener alternative to classical cross coupling reactions. This procedure has been described by Derridj et al in 200916.
To address this challenge, these substances were synthesized by palladium catalysis, using a new organic synthesis method. It allows single-step access to mono-bi and poly-dentate heterocyclic ligands that are more environmentally friendly.This simple and economical procedure allows access to heterocyclic ligands useful in the coordination chemistry17 (Fig. 2).
Materials and solutions
In this work we used mild steel with the following chemical composition (in wt%): 99.21% Fe; 0.21% C; 0.38% Si; 0.09% P; 0.01% Al; 0.05% S and 0.05% Mn. We prepared rectangular samples of the mild steel with the dimensions 2 x 2 x 0.3 cm as an electrode for the weight loss measurements. They were polished with SiC emery paper (60, 180, 240, 400, 600 and 1200), thoroughly rinsed with bidistilled water and dried by hot air.The aggressive solution was prepared from the dilution of 37% HCl analytical grade with bidistilled water18.
Results and discussion
Weight loss tests
Effect of concentration
The weight loss measurements were performed on mild steel samples immersed for 6 h in HCl (1 M) at 308 K, in the absence and presence of benzoxazol as inhibitors. This method is easy to handle and requires only simple equipment. Its principle is based on measuring the weight loss (Δm) of the mild steel sample with a total surface (S), during an immersion time (t) in a corrosive solution maintained at a constant temperature. The corrosion rate was described by the following relation:
where Wcorr is the corrosion rate in mg/h cm2, Δm is the weight loss in mg, S is the total surface in cm² and t is the immersion time in h.
The value of the inhibition efficiency was given as follow:
where Wcorr and Wcorr(inh) are the corrosion rates of steel in the absence and presence of the organic compound, respectively.
The values of EWL (%) for three inhibitors are gathered in Table 1.
From the results in Table 1 and Figure 3, we notice that the inhibition efficiency increases with higher concentrations of the inhibitor, whereas the corrosion rate decreases with the increase in L1, L2 and L3.
The results show that these compounds have a good inhibition efficiency in the studied medium and that their efficiencies reach 94%, 92% and 88% for L2, L3 and L1, respectively.
We also noted that the addition of the benzene group on the benzoxazole increases the effectiveness of steel inhibition corrosion due to the increased delocalization of the electron density in the molecule, which makes the compound more stable by the mesomeric effect19.
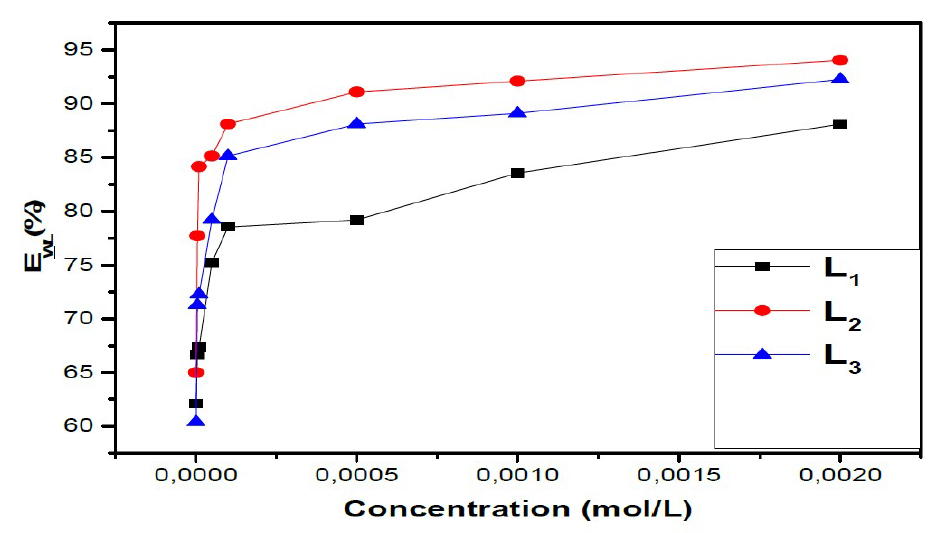
Figure 3 Variation of inhibition efficiencies with inhibitor concentration for mild steel in 1 M HCl at 308 K.
Effect of temperature
Temperature plays an important role in understanding the inhibitive mechanism of the corrosion process. To assess the effect of temperature on the corrosion and corrosion inhibitive process, weight loss experiments were performed in the temperature range of 308-348 K in uninhibited acid (1 M HCl) and inhibited solutions containing L1, L2 and L3 (210-3 M).
The corrosion rates and the inhibitory efficiencies of these compounds, as a function of temperature, are given in Table 2.
Table 2 Various corrosion parameters for mild steel in HCl solution (1 M) in the absence and the presence of L1, L2, L3 at different temperatures and optimum concentration after 1 h.
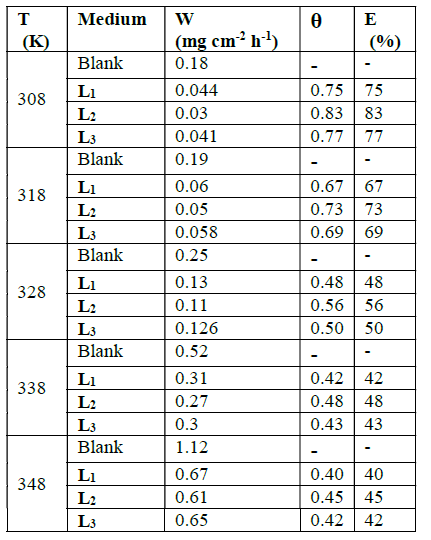
In this table we observe an increase in corrosion rates, in the presence and absence of inhibitors, with temperature. Inhibitory efficiency decreases with temperatures between 308 and 348 K. The evolution of the corrosion depends on the nature and structure of the molecules.
Thermodynamic activation parameters
Several studies20 have shown that the decrease in activation energy (Ea), during the addition of the inhibitor to an acidic solution, is attributed to the growth of the metal surface covered by the inhibitor molecule with increasing temperature. Therefore, the rate of corrosion depends on the temperature, according to the Arrhenius equation:
where k is the pre-exponential factor, T is the absolute temperature and R is the universal gas constant. Taking the logarithm of the Arrhenius equation21:
The plot of Ln W = f (1000/T) (Figure 4) gives a straight line of which slope (-Ea/R) allows us to calculate the activation energy (Ea) in the absence of the inhibitor which is of the order of 56.71 kJ mol-1 and which represents the energy barrier that the inhibitor has to cross to be adsorbed onto the surface of the plate.
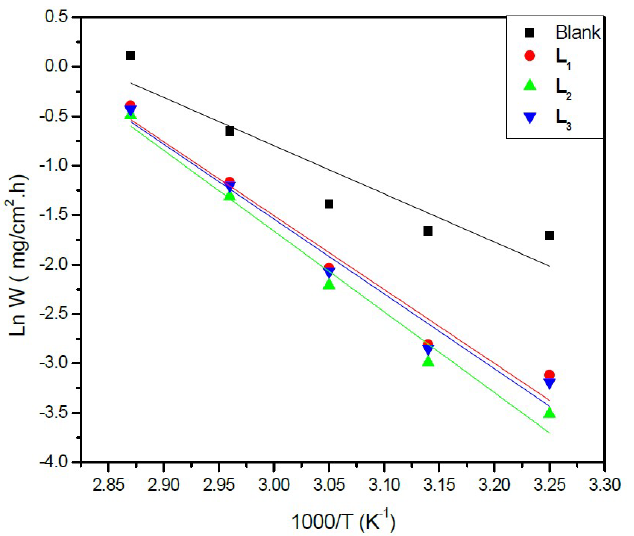
Figure 4 Arrhenius plots of Ln (w) vs (1000/T) for steel in a HCl solution (1 M) in the absence and the presence of L1, L2 and L3 at optimum concentration.
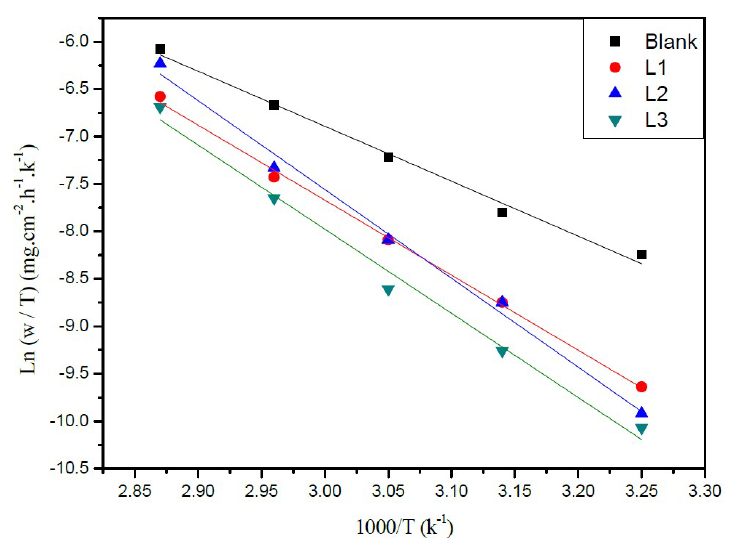
Figure 5 Arrhenius plots of Ln (w/T) vs. 1000/T for steel in a HCl solution (1 M) in the absence and the presence of L1, L2 and L3.
This result is in accordance with the values given in the literature for iron and steel in a HCl solution22. To access the thermodynamic activation characteristics (enthalpy (ΔHa °) and activation entropy (ΔSa °)), we used the Arrhenius transition equation23:
where h is the Plank constant and N is the Avogadro number.
Similarly, Figure 5 shows the variations of Ln (W/T) as a function of 1000/T under form of lines with a slope of -ΔHa ° / R and the intersection with the ordinate axis is [Ln (R / Nh) + (ΔS a ° / R)]. Aditionally, the values of ΔHa ° and ΔSa ° can be calculated (Table 3).
Table 3 Values of activation parameters Ea , Ha ° and Sa ° for mild steel in 1 M HCl in the absence and presence of different concentrations of L1, L2 and L3.
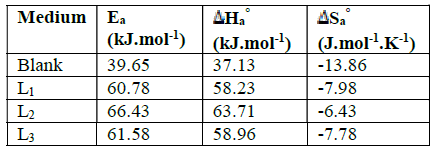
The activation energy value, Ea, obtained for L2 is higher than those obtained for the other investigated compounds, thus confirming the better inhibitory efficiency of L2.
The positive sign of enthalpy, Ha
°, indicates the endothermic nature of the steel dissolution process, while the negative entropy,
Sa
°, reflects the formation of a stable layer of inhibitor on the steel surface23.
Thermodynamic parameters of adsorption.
Attempts were made to fit surface coverage, θ, to different isotherms including Langmuir, Temkin Frumkin and Freundlich. The organic compound seems to follow well the Langmuir adsorption isotherm written in the rearranged form24:
where C is the inhibitor concentration, K is the adsorptive equilibrium constant, and ΔG°ads is the standard adsorption free energy.
The relationship between C/θ and C presents a linear behaviour at all studied temperatures (Fig. 6) with slopes equal to unity.
Table 4 thermodynamic parameters for the adsorption of L1, L2, L3 in 1 M HCl on the mild steel at 308 K.
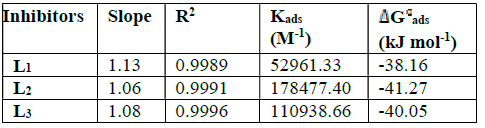
From the results given in Table 4, we can conclude that:
The adsorption isotherms allowed us to determine the thermodynamic parameters. The Linear regression coefficient was used to select the suitable isotherm (Table 4). It has been found that the linear regression coefficients are close to unity and all slope are close to 1.
The high values of adsorption equilibrium constants, Kads, reflect the high adsorption capacity of these inhibitors onto the mild steel surface. So, the Kads value of L2 is superior to those of other studied compounds. Such result suggests that L2 promotes the best recovery of the mild steel surface in comparison with other inhibitors, and, hence, its greater effectiveness in protecting against corrosion25,26.
The negative value of the free standard energy of adsorption (G
ads) indicates the spontaneity of the adsorption process of our molecules onto the steel surface27,28 and the stability of the formed layer which is due to the strong interaction between the inhibitory molecules and the metal surface29,30.
Generally, the standard free energy of -20 kJ.mol-1 or less is associated with the electrostatic interaction between the charged molecules and the charged metal surface (physical adsorption); those of -40 kJ.mol-1 or more negative implie a charge or transfer of inhibitory molecules to the metal surface to form covalent bonds with high binding energy (chemisorption)31. In this case, the adsorption of L1, L2 and L3 is of the chemical type.
Polarization potentiodynamic results
The potentiodynamic polarization curves were conducted using an electrochemical measurement system, PGZ 100 Potentiostat/Galvanostat, controlled by a PC supported by the Voltamaster 4.0 software. The electrochemical measurements were performed in a conventional three electrode glass cell with mild steel as a working electrode, platinum as auxiliary electrode (Pt) and a saturated calomel electrode used as reference electrode. The working electrode surface was prepared as described above in the gravimetric section. Prior to each electrochemical test, an immersion time of 30 min was given to allow the stabilization system at corrosion potential. The polarization curves were obtained by changing the electrode potential automatically from -800 to -200 mV/SCE at a scan rate of 1 mV s-1. The temperature is thermostatically controlled at a desired temperature of ±1K.The percentage protection efficiency, ETafel (%), is defined as:
where Icorr and Icorr(inh) are the corrosion current densities for the steel electrode in the uninhibited and inhibited solutions, respectively32.
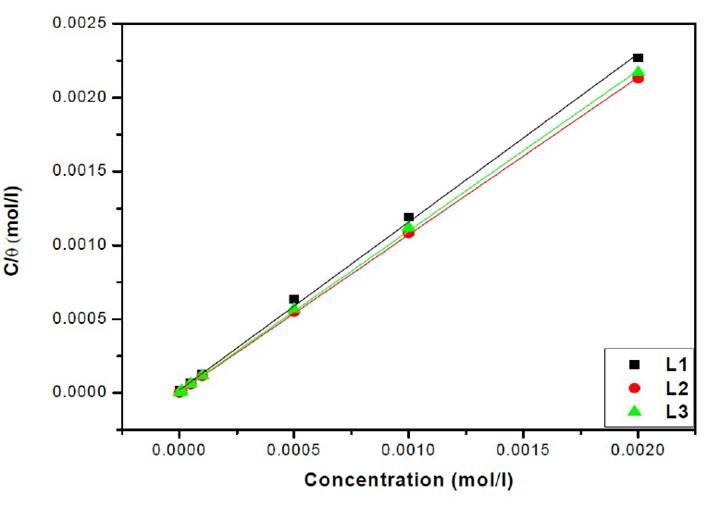
Figure 7a Polarisation curves of the mild steel in 1 M HCl for various concentrations of L1 at 308 K.
Figs. 7a, 7b and 7c represent the potentiodynamic polarization curves for carbon steel in 1.0 M HCl, in the absence and presence of various concentrations of this inhibitor.Table 5 shows the electrochemical corrosion parameters, i.e., the corrosion potential (Ecorr), cathodic and anodic Tafel slopes (βc, βa), corrosion current density (Icorr), obtained by extrapolation of the Tafel lines, and the inhibition efficiency (ETafel %).
Table 5 Polarization data of the mild steel in 1 M HCl, without and with addition of inhibitors, at 308 K
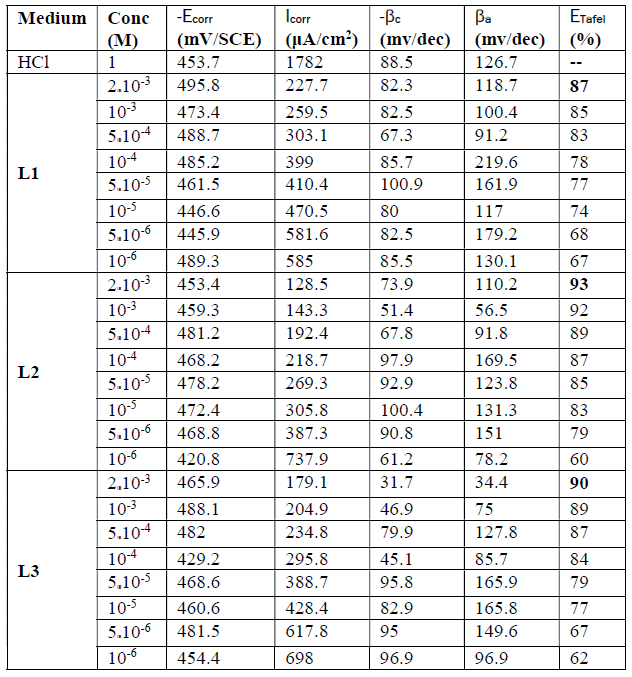
Examination of the results in Table 5 shows that the corrosion current density decreases as the concentration of the tested products increases and this is consistent with the increase in the inhibitory efficiency of our inhibitors proportionally to their concentration.
In the anodic domain, we note that the presence of L1, L2 and L3 results in a decrease in oxidation current densities. According to the literature45, an inhibitor could be classified as of the mixed-type when the change in the values of the corrosion potential, Ecorr, is lower than 85 mV. In our case, the largest displacement exhibited by the inhibitors was 40 mV; this result clearly indicates that these inhibitors have a cathodic and anodic effect. In addition, the βa and βc values of each inhibitor were found to change with the inhibitor concentration. Therefore, they can be classified as mixed inhibitors in a hydrochloric medium (1 M).
The addition of the compounds L1, L2 and L3 has no remarkable influence on the Tafel slope, in comparison with the results obtained in the case of the acid alone. As a result, the hydrogen reduction reaction at the steel surface is not modified and is carried out by a pure activation mechanism33, and the inhibitors first adsorb onto the steel surface, before acting by simply blocking its active sites.
Electrochemical Impedance Spectroscopy (EIS)
In order to confirm the results extracted from the polarization curve and to gain more information on the corrosion mechanisms, electrochemical impedance spectroscopy (EIS) measurements were carried out with sinusoidal potential waves of 10 mV amplitude, with frequencies ranging from 100 kHz to 10 mHz at the corrosion potential. The impedance diagrams are given in the Nyquist representation. The charge transfer resistance (Rct) was determined from Nyquist plots and the double layer capacitance (Cdl) was calculated from the constant phase element (CPE) parameters of the equivalent circuit determined by using Zview software. In this case, the percentage protection efficiency (Ez %) is calculated from the charge transfer resistance (Rct):
where and
are the charge transfer resistance in presence and in absence of the inhibitor, respectively.
Double-layer capacitance (Cdl) values were calculated using the following equation34:
where Fmax is the frequency at which the imaginary component of the impedance is greatest. The semicircle corresponds to a capacitive loop in the obtained electrochemical impedance diagrams. Deviations from a perfectly circular shape indicate the frequency dispersion of the interfacial impedance35.
The EIS diagrams of mild steel in 1 M HCl in the presence of L1, L2 and L3 at different concentrations are shown in Fig. 8aa, 8b and 8c, respectively.
The electrochemical parameters and the inhibitory efficiency for benzoxazole derivatives at different concentrations obtained from EIS measurements are gathered in Table 6.
The analysis of Table 6 shows that the values of Rct and inhibitory efficiency, EZ (%), become more important with an increasing concentration of the three benzoxazol derivatives. The double layer capacity, Cdl, decreases with a raise in the inhibitor concentration; this is due to the inhibitor molecules adsorption onto the metal surface, causing the decrease in their active surface. Therefore, the more the inhibitor is adsorbed, the more the thickness of the organic deposit is greater and, simultaneously, the double layer capacity decreases according to the expression of Cdl presented in Helmotz model:
where e is the thickness of the deposit, S is the electrode surface, ε0 is the permittivity of the medium and ε is the dielectric constant. This change in Rct and Cdl is probably due to the displacement of water molecules by the Cl- ions of the acid and to the adsorption of organic molecules onto the metal surface, thus decreasing the metal dissolution rate and reduction of H+ protons36.
Quantum chemical calculations
To correlate the experimental data obtained from the weight loss, the quantum indices of L1, L2 and L3 are computed using B3LYP/6-31G (d, p). For this purpose, the program Gaussian 09 w37 was used with an optimization of the geometry.
According to the frontier molecular orbital theory (FMO) of chemical reactivity, the transition of electrons is due to the interaction between the highest occupied molecular (HOMO), the lowest unoccupied molecular orbital (LUMO) of the reacting species38 corrosion and EHOMO, which is often associated with the electron-donating ability of the molecule. The literature shows that the adsorption of the inhibitor onto the metal surface can occur on the basis of donor-acceptor interactions between the π-electrons of the heterocycle compound and the vacant d-orbitals of the metal surface atoms39. The energy of ELUMO indicates its ability to accept electrons. The high values of EHOMO are likely to indicate a tendency of the molecule to donate electrons to appropriate acceptor molecules with low energy and empty molecular orbital. A low E value should facilitate the adsorption of the molecule and therefore cause an increase in the inhibitory efficiency40. According to Koopmans' theorem41, the energies of HOMO and LUMO of the inhibitors are related to the ionization potential, I, and the electronic affinity, A, by the following relations:
The absolute electronegativity (χ), and the absolute hardness (η) of the inhibitor molecule are given by42.
The fraction of transferred electrons (N), non-binding doublets of the nitrogen atom and / or electrons π, to the d orbital of the Fe atom is given by40:
In order to calculate the fraction of transferred electrons (N), a theoretical value of the electronegativity of iron equal to
Fe = 7eV and an overall hardness (Fe = 0 were used40. Among the calculated parameters, we cite the HOMO energy of EHOMO, LUMO energy of ELUMO, HOMO-LUMO energy gap, ΔEgap, and dipole moment, µ. The quantum-chemical parameters in the neutral form are given in Table 7.
The optimized minimum energy structure of L1, L2 and L3 is shown in Fig.9.
The results presented in Table 7 show that the energy difference is lower, 0.15252 eV; therefore, the high reactivity of the inhibitor can allow it to be easily adsorbed onto the mild steel surface leading to an increase of its inhibitory efficiency.
The dipole moment given in Table 7 is the measurement of the polarity inside the whole molecules43. It is usually an index used for predicting the direction of corrosion inhibition. Therefore, it is generally accepted that the adsorption of polar compounds with high dipolar moments onto the mild steel surface should lead to a better inhibition efficiency44. The results show that L2 has the highest dipole moment, 2.6510 (debye). Therefore, we can assume that the adsorption of L2 onto the metal surface will be stronger than those of L1 and L3 and therefore the effectiveness of the corresponding inhibition will follow the order μ (L1) <μ (L3) <μ (L2). These results are in good agreement with the experimental results.
The hardness reflects the resistance of a system to changing its number of electrons. A low hardness means that it is easy for the molecule to yield or capture the electrons: η (L2) <η (L3) <η (L1).
The higher the number of electrons transferred, the better the adsorption onto the metal surface is promoted: ΔN (L1) <ΔN (L3) <ΔN (L2). These results may lead to an increased adsorption onto the metal surface and increase the efficiency inhibitors accordingly. In relation to experimental inhibitory efficiency, all descriptors correlate with inhibitory efficiencies.
In relation to the experimental inhibitory efficiency, the calculated descriptors are in good correlation with the inhibitory efficiency for the L2 molecule which has the highest efficiency (94%).
Conclusions
This work was devoted to the study of organic benzoxazole inhibitors on the corrosion of mild steel in a 1 M hydrochloric acid medium. This study was carried out by gravimetric and electrochemical methods, both stationary and transient. We can conclude that the studied products are good inhibitors and that their efficiencies reach maximum values at 2.10-3 M. The inhibitory power of the latter follows the order: L2 >L3 >L1.
The adsorption of inhibitors L1, L2 and L3 onto the steel surface follows the Langmuir isotherm. The negative values of ΔG°ads indicate that the absorption of these molecules in the corrosive medium occurs spontaneously onto the steel surface.
The comparative study of the different used methods (gravimetry and methods electrochemistry) shows a very good concordance of the results which confirms the complementarity of these two methods.
Theoretical calculations show that molecules with a small energy gap and a high dipole moment exhibit good inhibitory efficiency.