Introduction
Activated carbon (AC) production is one of the most important challenges for commercial manufacturers, and using inexpensive raw materials with high carbon content and low levels of inorganic compounds to produce low cost AC has been a focus of research efforts in recent years. Agricultural by-products and waste material such as rice husks (1), coconut husks (2) and oil palm fibers (3) are among the low-cost precursors for the production of AC.
Steam pyrolysis is the simplest and most economic method for the production of AC. Steam pyrolysis is a physical activation process in which the precursor material is heat-treated in the presence of steam to form a porous carbonaceous char. Physical activation involves two-steps carbonization of the carbonaceous raw material to a carbonaceous char, and activation of the char in the presence of a suitable activating medium such as carbon dioxide, steam or air.
Carbonization occurs between 400 and 800 °C, while activation occurs between 600 and 900 °C (4). Over the last several years, many promising results have been obtained by using a microwave irradiation method for the preparation of relatively homogeneous inexpensive AC particles with high surface area and significant adsorption capacity. The use of microwave radiation causes higher sintering temperatures, shorter processing times and, therefore, higher energy savings (5).
Chemical and physical activation are two types of activation processes used in the preparation of AC. Physical activation is the partial gasification of the carbonaceous material, where this is carbonized at high temperatures in a furnace under an inert atmosphere, such as nitrogen, to eliminate most of the hydrogen and oxygen content and produce char with the desired porosity. Chemical activation involves mixing an acidic or basic solution with the carbonaceous material to influence the pyrolytic decomposition of the starting materials and lower the pyrolysis temperature. Various technologies have been applied recently to remove toxic components such as anions, heavy metals, organic compounds and dyes from water sources (6-8). Adsorption technology is one of the applicable simple methods for water treatment (9).
AC is used as a potential adsorbent in processes such as the purification of industrial effluents (10), groundwater treatment (11) and the removal of volatile organic compounds from air and mercury vapors from a gas mixture (12). AC has demonstrated significant adsorption in gas and liquid phases due to its high micro pore volume (Vmic), large specific surface area (SBET), favorable pore size distribution, thermal stability, capability or rapid adsorption and low acid/base reactivity (13).
In this paper, the adsorption capacity of AC (prepared from sawdust of beech wood and its amide derivatives) towards Ni(II), Zn(II) and Co(II) from aqueous solutions was studied varying treatment conditions such as contact time, weight of sorbent, pH and initial concentration of treated metal ions.
Experimental
Adsorbent material
The adsorbent material is the activated carbon prepared from sawdust of beech wood and its amide derivatives; sawdust of beech wood was collected from its origin sites. The samples collected from each location were transported to the laboratory and stored in the desiccators within the limited time prior to the physical and adsorption characterizations.
Chemicals
All chemicals used were high-grade chemicals from Merck, BDH and Fisher companies: buffer solutions of pH equal to 1, 2, 3, 4, 5 and 6 for calibration of the pH-meter; concentrated phosphoric acid (63%); an individual stock standard solution of zinc chloride (ZnCl2); an individual stock standard solution of nickel chloride (NiCl2); an individual stock and a resolution of hydrated cobalt chloride (CoCl2.6 H2O); nitric acid (70%) and sulphuric acid (98%); phosphorus pent chloride; sodium hydroxide pellets; acetic anhydride; and acetone.
Preparation of AC
Sawdust of beech wood, a cheap material, was chosen as a precursor for the preparation of AC by one step chemical activation using concentrated H3PO4 (63%) (14). In this concern, a known weight of the crushed sawdust of beech wood was soaked in 63% (w/w) of H3PO4.The volume of H3PO4 should be enough to cover the sawdust completely, and the system was slightly agitated to ensure complete penetration of the acid throughout the sawdust. The mixture was heated to 110 °C for one hour, and left over night at room temperature, to help appropriate wetting and impregnation of the precursor. The impregnated mass was dried in an air oven at 110 °C overnight, then it was placed in an electric furnace, where the temperature was raised at a rate of 10 °C/min up to 400 °C, and then it was left for 4 hours. The product was washed thoroughly with hot distilled water until pH=5, then it was dried at 110 °C, and finally it was grinded and sieved using a 60 mesh sieve.
Preparation of AC with amide group
Preparation of carboxylic acid derivatives from AC
The prepared activated carbon was immersed in acetone, then filtered and immersed again in 0.1 N NaOH for an hour, under stirring at 25 °C (15). The mixture was filtered and washed with distilled water several times until a neutral solution was obtained, and then it was dried at 100 °C for an hour. The obtained mixture was the functionalized carboxylic group (COOH), by refluxing with a mixture of nitric acid (70%) and sulphuric acid (98%), at a ratio of 1:3 at 160 °C, for 90 min. The mixture was cooled, washed and dried at 100 °C, for 24 hours.
Preparation of acetyl derivatives
The conversion of carboxylic acid into the corresponding acyl chloride (16) is usually achieved by heating the acid with phosphorus pentachloride (scheme 1). A 100 mL two necked flask was fit with a dropping funnel and a reflux condenser connected at the top to a gas absorption trap. 36 g of phosphorus pentachloride and 22 g of the prepared carboxylic derivative were placed in the flask. The flask was gently heated on a water bath for 30-40 min. The apparatus was rearranged, and the mixture was filtered, then dried at 100 ± 5 °C.
Preparation of amide derivatives
0.5 g of ethyl amine in 2 M hydrochloric acid were mixed with the prepared acetyl derivative (17), and a little crushed ice was added. A solution of 5 g of sodium hydroxide was introduced in 25 mL of water, followed by 5 mL of acetic anhydride. The mixture was shaken in the cold until the smell of acetic anhydride disappeared. The solid acetyl derivative was collected, and recrystallized from water;
where R is activated carbon and R΄ is ethyl group (- C2H5).
Determination of heavy metal ions
In flame atomic absorption, a sample was aspired into a flame and atomized. A light beam was directed through the flame into a monochromator, and onto a detector that measures the amount of light absorbed by the atomized element in the flame.
For some metals, atomic absorption exhibits superior sensitivity over flame emission. Because each element has its own characteristic absorption wavelength, a source lamp composed of that element was used, which is called the Hollow Cathode Lamp.
This makes the method relatively free from spectral or radiation interference. The amount of energy at the characteristic wavelength absorbed in the flame is proportional to the concentration of the element in the sample over a limited concentration range.
The instrument used for the determination of heavy metals concentration was an AA-6800 - SHIMADZU atomic absorption spectrophotometer.
The method used for the determination of heavy metals was 3111B Direct Air-Acetylene Flame Method, edition 19 - 1995, Standard Method for the Examination of Water and Wastewater.
Factors affecting adsorption of heavy metal ions
Effect of weight of activated carbon on the removal of heavy metal ions
A multi-element standard solution containing Ni(II), Zn(II) and Co(II) metals, which concentration was equal to 100 mg/L, was prepared. The pH of the standard solution was adjusted to 6.5. To a 50 mL of the multi-element standard, 0.1, 0.2, 0.3 and 0.4 g of the activated carbon were put in an Erlenmeyer flask, and the mixture was shacked using a rotary shaker at about 100 rpm for 2 hours. After that, the mixture was filtered using a 0.45 µm filter paper. The filtrate and the multi-element standard were analyzed using an atomic absorption spectrophotometer.
Effect of time on the removal of heavy metal ions
A multi-element standard solution was prepared previously; to 50 mL of each solution, 0.4 g of the activated carbon were added, and the mixtures were shacked for 30, 60, 90 and 120 min, and were analyzed by using an atomic absorption spectrophotometer.
Effect of pH on the removal of heavy metal ions
The prepared standard solutions, which had different pH values (1, 2, 3, 4, 5 and 6) were treated as previously and analyzed using an atomic absorption spectrophotometer.
Effect of initial concentration on the removal of heavy metal ions
A series of multi-element standard solutions which concentrations were equal to 20, 60 and 100 mg/L were prepared. The solutions were treated as previously, and they were analyzed using an atomic absorption spectrophotometer.
Results and discussion
Analysis of lignocellulosic materials (Table 1)
Characterizations techniques of bio-sorbents (Table 2)
Table 2 Acid-water content and pH for different types of AC.
AC prepared from beech wood | AC with amide group | |
---|---|---|
Solubility in 0.2 M HCl (%) Water solubility (%) pH | 2.03 0.026 2.03 | 2.23 0.032 6 |
Infrared spectra of activated carbon and of activated carbon with amide group
FT-IR technique was used to identify the functional groups that are present in activated carbon and activated carbon with the amide group. The spectra of activated carbon (Fig.1a) showed bands in the region ranging from 1025 to 1159 cm-1, indicating the presence of -O-H groups. An additional characteristic adsorption band appeared at 2850 cm-1, for C-H stretches. The -CH stretching in the methylene group was detected at 2923 cm-1. A broad band due to hydrogen bonded -OH groups appeared at 3423 cm-1. A small sharp peak at 669 cm-1, which was attributed to C=C symmetry bands, reduced intensity.
IR spectrum of activated carbon with the amide group (Fig.1b) exhibited the new absorption band at 1649 cm-1, which was attributed to the carbonyl group (C=O) of the amide group. The OH band and C-O-C band intensities were at 3423.81 cm-1 and 1096 cm-1, respectively. A small sharp peak at 3333 cm-1indicated the presence of the -NH group, and possibly also of moisture. Bands ranging from 1210 to 1320 cm-1 and 1703 cm-1 are related to COOH derivatives.
Sorption studies
Effect of weight of activated carbon on the removal of the heavy metal ions
The experiments were carried out with 0.1, 0.2, 0.3 and 0.4 g of the activated carbon which were added to a prepared standard solution (synthetic wastewater).
The effect of the weight of the sorbent (activated carbon) on the removal percentage of Ni(II), Zn(II) and Co(II) ions is shown graphically in Fig. 2. Inspection of the data obtained showed that the removal percentages were enhanced by increasing the weight of the sorbent for all heavy metals, but with different percentages, as follows:
- maximum removal percentage was obtained for Ni(II) ion (0.4 g of sorbent /50 mL of the metal solution), which is nearly equal to 99.2 % and is nearly independent of the weight of the used sorbent.
- when using 0.1 g of sorbent/50 mL of metal solution, minimum removal was obtained for Ni(II) ion, which is nearly equal to 89.36 %.
- the variation of maximum removal percentage of metal ions with weight of activated carbon used as sorbent lies in the order of Ni(II) < Co(II) < Zn(II), corresponding, respectively, to 99.2, 95.02 and 92 %.
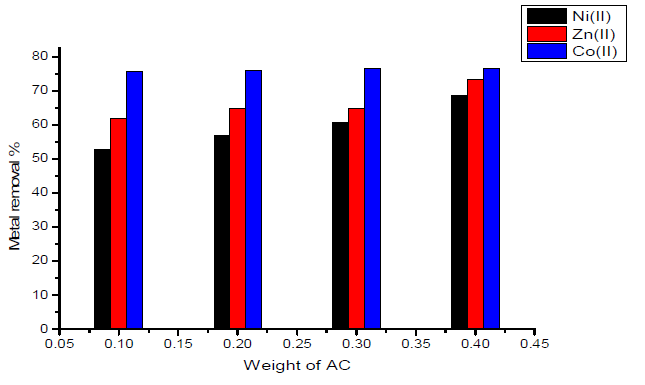
Figure 3 Effect of the weight of activated carbon with amide group on the removal of heavy metal ions.
The effect of weight of the sorbent (activated carbon with amide group) on the removal percentage of Ni(II), Zn(II) and Co(II) ions is shown graphically in Fig. 3. Inspection of the data obtained showed that:
- maximum removal percentage was obtained for Co(II) ion, which is nearly equal to 76.7%.
- when using 0.1 g of sorbent, minimum removal was obtained for Ni(II) ion, which is nearly equal to 52.8%.
- the variation of maximum removal percentage of metal ions with weight of activated carbon used as sorbent lies in the order of Co(II) < Zn(II) <Ni(II).
Effect of contact time on the removal of heavy metals
Studies on the effect of contact time on the maximum removal of the metal ions under investigation showed that the removal percentage is enhanced at an increased contact time with the sorbent, and reaches its maximum value after 120 min. The results are shown in Fig. 4.
The effect of contact time in the case of activated carbon with amide group is showed in Fig. 5.
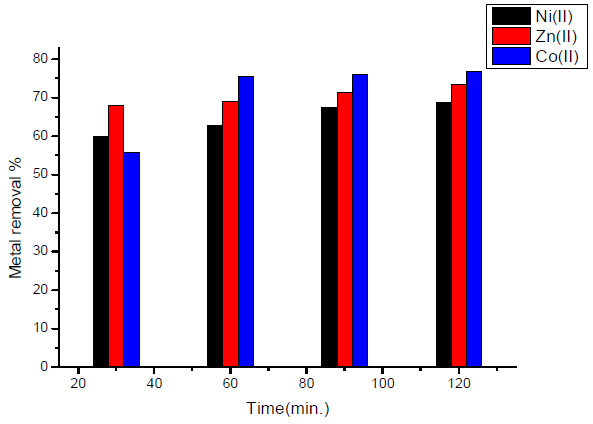
Figure 5 Effect of contact time, in the case of activated carbon with amide group, on the removal of heavy metal ions.
Effect of pH on the removal of heavy metals
Results that are presented in Fig.6 showed that maximum removal takes place at pH 5, which indicates that maximum adsorption affinities take place in a moderate and slight acidic media. Co(II) ion shows a notable trend to be adsorbed onto the activated carbon in strong, moderate and slight acidic media.
In the case of activated carbon with amide group, Fig.7 showed that maximum adsorption takes place at pH equal to 6 for Zn(II) ion.
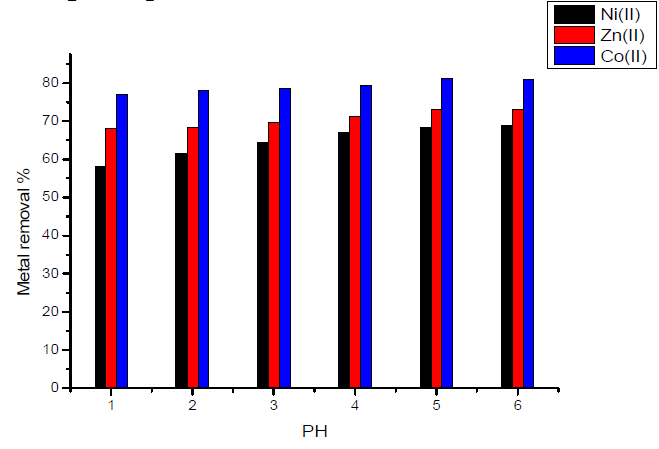
Figure 7 Effect of pH on the removal of heavy metal ions, in the case of activated carbon with amide group.
Effect of initial concentration on the removal of heavy metals
The removal percentage with change in the initial concentration of heavy metals showed that: maximum adsorption at initial concentration (100 mg/L) was obtained for Ni(II) ion, which is nearly equal to 99.2 %; and minimum adsorption at the initial concentration (100 mg/L) was obtained for Zn(II) ion, which is nearly equal to 92 %.The results are shown in Fig.8.
In the case of activated carbon with the amide group, maximum adsorption at initial concentration (100 mg/L) was obtained for Co (II) ion, which is nearly equal to 76.7%, and minimum adsorption at an initial concentration (100 mg/L) was obtained for Ni (II) ion, which is nearly equal to 15%.The results are shown in Fig.9.
Adsorption isotherms
Adsorption data for adsorbate concentrations are most commonly described by an adsorption isotherm, such as the Langmuir, Freundlich or Temkin isotherms.
The Langmuir isotherm is valid for monolayer adsorption onto a surface containing a finite number of identical sites. The model assumes uniform energies of adsorption onto the surface and no transmigration of the adsorbate in the plane of the surface. It is represented by the following equation:
where Ce is the concentration of the metal ion solution (mg/L) at equilibrium, Qo signifies the adsorption capacity (mg/g) and b is related to the energy of adsorption capacity (mg/g). A linear plot of Ce/qe versus Ce shows that adsorption follows Langmuir isotherm. Qo and b (Fig. 10) were calculated from the slope and intercept of the linear plots which are present in Table 3.
Table 3 Langmuir adsorption isotherm parameters of metal ions onto activated carbon.
Metal ion | R2 | Slope | Intercept |
---|---|---|---|
Ni(II) | 0.62 | 0.01 | -0.36 |
Zn(II) | 0.92 | 3.45 | -0.24 |
Co(II) | 0.85 | 2.42 | 0.095 |
In the case of activated carbon with the amide group, adsorption follows Langmuir isotherm (Fig.11). Qo and b were calculated from the slope and intercept of the linear plots and are present in Table 4.
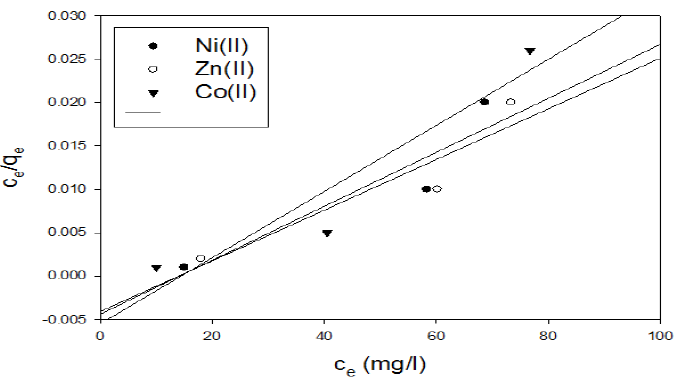
Figure 11 Langmuir isotherm plot for the adsorption of metal ions onto activated carbon with the amide group.
Table 4 Langmuir adsorption isotherm parameters of metal ions onto activated carbon with the amide group.
Metal ion | R2 | Slope | Intercept |
Ni(II) | 0.87 | 0.0031 | -0.049 |
Zn(II) | 0.88 | 0.0029 | -0.041 |
Co(II) | 0.90 | 0.0038 | -0.055 |
The Freundlich adsorption isotherm was also applied for the adsorption of metal ions on activated carbon. The Freundlich equation is represented as:
efor
efSo, by plotting log qe vs. log C, the constant Kf and the exponent (n) can be determined. Freundlich linear relation is shown in representative examples in Fig.12. The slope and intercept of the linear plots are present in Table 5.
Table 5 Freundlich adsorption isotherm parameters of metal ions on activated carbon.
Metal ion | R2 | Slope | Intercept |
---|---|---|---|
Ni(II) | 0.62 | -2.227 | 16.2 |
Zn(II) | 0.95 | -5.71 | 32.7 |
Co(II) | 0.88 | -2.33 | 17.42 |
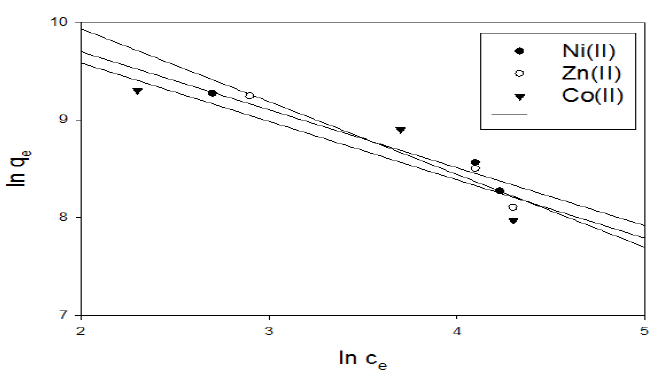
Figure 13 Freundlich isotherm plot for adsorption of metal ions onto activated carbon with the amide group.
In the case of activated carbon with the amide group, Freundlich linear relation is shown in representative examples in Fig. 13. The slope and intercept of the linear plots are present in Table 6.
Table 6 Freundlich adsorption isotherm parameters of metal ions on activated carbon with the amide group.
Metal ion | R2 | Slope | Intercept |
---|---|---|---|
Ni(II) | 0.96 | -0.59 | 10.88 |
Zn(II) | 0.95 | -0.74 | 11.4 |
Co(II) | 0.81 | -0.59 | 10.76 |
Temkin isotherm is represented by the following equation:
eeThis equation can be expressed in its linear form as:
e11ewhere B1=RT/b.
The adsorption data can be analyzed according to the previous equation. A plot of qe versus lnCe enables the determination of the KT and B1 isotherm constants in Fig. 14. The slope and intercept of the linear plots are present in Table 7.
Table 7 Temkin adsorption isotherm parameters of metal ions onto activated carbon.
Metal ion | R2 | Slope | Intercept |
Ni(II) | 0.96 | -5748.9 | 27380.5 |
Zn(II) | 0.99 | -11701.9 | 53719.3 |
Co(II) | 0.98 | -6973.6 | 32992.1 |
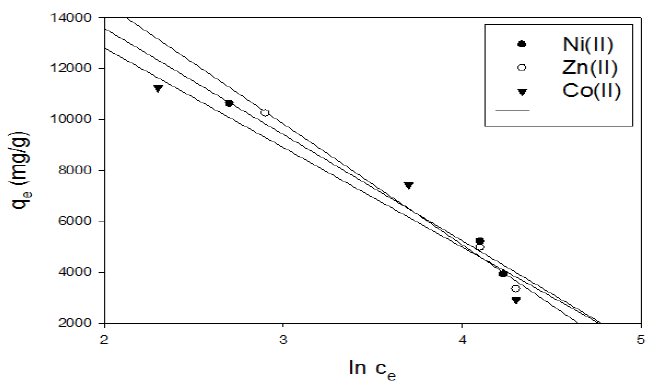
Figure 15 Tempkin isotherm plot for adsorption of metal ions onto activated carbon with the amide group.
In the case of activated carbon with the amide group, a plot of qe versus lnCe enables the determination of the KT and B1 isotherm constants in Fig. 15. The slope and intercept of the linear plots are present in Table 8.
Conclusion
Based on the present investigation, it could be concluded that some low cost materials such as activated carbon prepared from sawdust of beech wood can be used efficiently in the removal of heavy metal ions (Ni(II), Zn(II) and Co(II)) from aqueous solutions. The removal of heavy metal ions was pH dependent, as the adsorption capacity was enhanced with increasing pH values of the solution and, at a particular pH, the order of increasing removal percentages was Zn(II) > Ni(II) > Co(II). The metal ions showed different behaviors towards adsorption onto activated carbon by increasing the initial concentration of metal ions. The control of the adsorption process was straightforward. The experimental studies showed that activated carbon prepared from sawdust of beech wood could be used as an alternative, inexpensive and effective material to remove high amounts of toxic heavy metal ions from wastewater. Comparing activated carbon and activated carbon with amide group, we found that the best conditions were achieved in the case of activated carbon which is prepared from sawdust of beech wood.