Introduction
When a metal comes in contact with its surroundings, it might undergo some chemical reactions, such as electrochemical oxidation with some oxidants such as oxygen; this leads to the phenomenon called corrosion (1. One of the important properties for an engineering material is its ability to resist corrosion 2.
Aluminum and its alloy are vital materials, because of their wide range of mechanical applications, particularly in aerospace and household industries. The wide use of aluminum and aluminum alloys is because of their principle properties like lightweight, formability, recyclability, solidness, ductility and conductivity. These special properties make them an important material 3.
Since aluminum is used in multiple industrial purposes, so, the study of its corrosion inhibition is much needed.
The overall cost and environmental implications of various problems associated with corrosion in many industries have led to several efforts adopted for reducing the risk of metals against corrosion 4.
A corrosion inhibitor can be defined as any substance which, when added to a metal, reduces its rate of corrosion. Due to their property of minimizing metallic waste, corrosion inhibitors are of great practical importance 5. The utilization of inhibitors is the most useful strategy to cope with this issue. Recently, organic and inorganic compounds have been normally added to HCl solution as corrosion inhibitors; however, some of these inhibitors are toxic, non-biodegradable and expensive to integrate.
In an attempt to discover corrosion inhibitors which are environmentally safe and readily accessible, there has been a growing trend in the utilization of natural products, for example, leaves or plants extract as corrosion inhibitors for metals in the corrosive cleaning process. This is because plants are the sources of naturally occurring chemical compounds that are acceptable, cheap, promptly accessible and renewable sources of materials 6,7.
Literature suggests that many plant extracts have been used as corrosion inhibitors. Some of them are; Cassia 8, caffeic acid 9, Cappari deciduas 10, Euphorbia hirta 11, Hibiscus rosa-sinensi 12, Hibiscus subdariffa 13, jojoba oil 14, red onion skin 15, Jasminum nudiflorum 16, Raphia hooker 17, bamboo leaf extract 18, Ginkgo leaves 19, henna extract 20, Artemisia pallens 21, Oxandra asbeckii 22, Neolamarckia cadamba 23, Telferia occidentalis 24, Sansevieria trifasciata 25, Oxandra asbeckii 26, Tulsi 27, Nipah 28, justicia gendarissa 29, and Andrographis paniculata plant extracts which have all showed maximum inhibition efficiency, up to 98%.
Considering the inhibition efficiency of the above mentioned plant extracts which is 80-90%, this encourages us to continue this pursuit with an aim to achieve higher efficiency. In the present investigation, an attempt has been made to study the inhibitive action of the plant extract, Gymneme Sylvestre, on the corrosion of aluminum in HCl.
The leaves of Gymnema sylvestre contain triterpene saponins, belonging to oleanane and dammarene classes, in which dammarene saponins are gymnemasides, while oleanane saponins are gymnemic acids and gymnemasaponins.
The leaves also contain chlorophyll, tartaric acid, carbohydrates, formic acid, resins, butyric acid, cellulose (22%), inositole alkaloids, organic acid (5.5%), albumin, calcium oxalate (7.3%), anthraquinone derivatives, lignin (4.8%) and parabin. The active constituents are gymnestogenin, gymnemic acid and gymnemanol 30. Many methods have been used to measure the inhibition efficiency of the inhibitor viz; weight loss, electrochemical studies, Tafel Polarization and quantum chemical studies 31.
Material and methods
Preparation of material
Gymnema sylvestre leaves were shade dried for 48 hours and were grinded to powder. 100 grams of this powder were used in a soxhlet apparatus, in order to make the aqueous extract of Gymnema sylvestre. This aqueous extract was then filtered and was tray dried for 48 hours at 318.15 K. The dried powder obtained after drying was preserved in desiccators. The test solutions of different concentrations were made using this dried extract in 0.5 M HCl as a medium.
Weight loss method
The aluminum coupons having a composition of 0.35% Fe, 0.25% Si, 0.05% Cu, 0.05% Zn, 0.03% Mg, 0.03% Mn, 0.03% Ti and the remainder 99.60% being Al were utilized for weight loss studies. The measurements of aluminum coupons utilized for weight loss studies were 2 × 2 cm2. Before performing the investigation, the coupons were degreased with emery papers (100-1500 grades); then these were washed with distilled water and acetone. The coupons were then dried for further utilization. The coupons were weighed before and after immersion in an acidic solution and in the inhibitor. Weight loss was used to calculate the inhibition efficiency using the following equation:
where IE (%) is the inhibition efficiency and Wo and Wt are the initial and final weight, respectively.
The different factors influencing the adsorption of the inhibitor are the nature of the metal and the charge that the metal carries, charge distribution in the molecule, chemical structure of the inhibitor, and the type of electrolyte.
Electrochemical studies
In order to know the mechanism of adsorption of the inhibitor of aluminum corrosion, the potentiodynamic studies were performed using CH-700 instrument, USA. Measurements were performed on aluminum immersed in an acidic solution with different concentrations of inhibitor (200, 400, 600 and 800 ppm) at 298K. Different parameters were elucidated viz., corrosion current density, corrosion potential and inhibition efficiency.
Results and discussion
Weight loss measurements
The weight loss data in the presence of inhibitor are shown in Table 1 and Fig. 1a. It was observed that, when we increase the concentration of inhibitor, its efficiency increases. In 0.5 M HCl, the corrosion rate of the aluminum coupon increases, reaching its maximum at 800 ppm concentration of the inhibitor. As the concentration of inhibitor increases, η % also increases, indicating that the inhibitor blocks the active sites, thus forming a protective layer.
Table 1 Weight loss measurements of corrosion inhibition of Al in 0.5 M HCl using Gymneme Sylvestre.
Inhibitor concentration (ppm) | Weight loss (mgcm-2) | η % | CR (mm/y) | Θ |
---|---|---|---|---|
Blank | 20.8 | - | 675 | - |
200 | 6.0 | 70.9 | 49.0 | 0.70 |
400 | 5.4 | 74.0 | 43.8 | 0.74 |
600 | 4.7 | 77.7 | 37.5 | 0.77 |
800 | 3.7 | 82.2 | 30.0 | 0.82 |
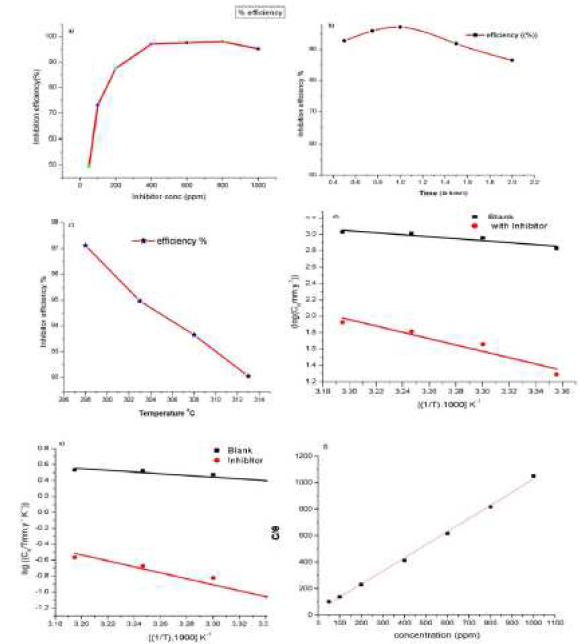
Figure 1 (a) Inhibition efficiency variation of Al in different concentrations of inhibitor in 0.5 M HCl, (b) Inhibition efficiency variation with immersion time, (c) Inhibition efficiency variation with different temperatures, (d) Arrhenius plot of corrosion rate CR vs. 1/T and (e)Transition state plot of CR/T vs. 1/T, (f) Langmuir adsorption isotherm plot of aluminum corrosion inhibition.
Effect of temperature
The effect of temperature on the corrosion of an aluminum sheet in the acidic medium was measured at temperatures ranging from 298 to 313 K. This shows that with an increase in temperature, the inhibitor efficiency decreases.
The log of corrosion rate is a linear function with 1/T (Arrhenius equation) 32.
where Ea is the activated energy, R is the gas constant and λ is Arrhenius pre exponential factor. The graph between log CR and 1/T gave an almost straight line in Fig. 1d, with the slope of -Ea/2.303R. The values of activation energy are shown in Table 2.
We can find out ΔH (enthalpy of activation) and ΔS (entropy of activation) by the following relationship.
Taking log on both sides we get:
The plot of log(CR/T) vs1/T, the straight line with the slope of -ΔH/2.303R shown in Fig.1e and the intercept of log(R/Nh) + ΔS/2.303R were obtained, which gives values of ΔS and ΔH that were determined (Table 2).
Table 2 . Thermodynamic parameters for aluminum sheet in 0.5M HCl in the presence and absence of inhibitor.
Inhibitor concentration (ppm) | Ea (kJmol-1) | ΔH (kJmol-1) | ΔS (Jmol-1K-1) | |
---|---|---|---|---|
Blank | 23.1 | 20.5 | -122 | |
800 | 74.0 | 71.5 | 21.0 | |
Temperature (K) | ΔG0 ads (kJ mol-1) | |||
298 | -37.6 | |||
303 | -23.9 | |||
308 | -18.9 | |||
313 | -12.2 |
From the observation of this data, positive sign of ΔH0 ads shows that the dissolution process is of an endothermic nature. The activation energy of the inhibited solution is greater than the blank solution, which suggests the formation of a protective layer on the metal surface. The standard Gibbs free energy of adsorption, ΔG0 ads, and thermodynamic parameters were used to determine the inhibitive mechanism. The equilibrium constant, Kads, at different temperatures, gave the value of ΔG0 ads by this equation:
In the above equation, 55.5 is concentration of water in the solution in mol/lit, R is the gas constant and T is the absolute temperature. The corresponding values of ΔG0 ads are given in Table 3. The adsorption of the inhibitor molecules on the aluminum coupon is spontaneous, which is indicated by the negative value of ΔG0 ads. The higher values of ΔG0 ads around -40 kJmol-1 indicate the formation of a coordinate bond between aluminum and the inhibitor molecules, giving rise to chemisorption; lower values of ΔG0 ads indicate electrostatic interaction or physisorption. In our experiment, the value of ΔG0 ads was observed to be from -12.16 to -37.68 33-34.
Adsorption isotherm
The corrosion inhibition mechanism was found to be out from the values of the adsorption isotherm. The surface coverage (θ) is given by equation.
where W0 = corrosion rate in the absence of inhibitor and W = corrosion rate in the presence of inhibitor.
The surface coverage (θ), concentration of inhibitor variation (C) and K are the adsorption constant and can be related to each other as:
Results showed that Langmuir adsorption isotherm is the best fit with our data. The graph between C/θ and C shows a straight line and a regression coefficient value near to 1 (R2 = 0.99983) in Fig. 1e.
Electrochemical impedance spectroscopy (EIS)
The electrochemical impedance spectroscopy was performed to understand the characteristics and kinetics of the electrochemical reactions occurring on the aluminum surface in the acidic solution. The adsorption of the inhibitor on aluminum causes a notable increase in the impedance at the interface, which means an increase in resistance to the charge transfer process. Therefore, the idea of inhibition performance of an inhibitor can come from EIS measurements. The impedance obtained in the presence and absence of the inhibitor was compared to knowing the degree of corrosion protection. The calculated value of electrochemical impedance spectroscopy is given below (Table 4).
Table 4 EIS parameters for corrosion of Al in 0.5 M HCl, in the absence and presence of acarbose, at 298K.
Conc.(ppm) | Rct | C(μF) | IE |
---|---|---|---|
Blank | 3.9 | ||
200 | 10.2 | 41358 | 61.7 |
400 | 16.2 | 8592 | 75.4 |
600 | 22.2 | 104308 | 86.6 |
800 | 29.5 | 148482 | 86.6 |
Fig. 2 (a) shows the Nyquist plots for aluminum in a 0.5 M HCl solution, with and without inhibitor, in the concentration range of 200 to 800 ppm, at 298 K. The impedance spectrum at each condition gives depressed capacitive imperfect semicircles in the high frequency region, which is credited to the charge transfer process and to the formation of a protective layer by the inhibitor on the metal surface. The imperfect semicircle nature of the impedance spectrum is credited to the mass transfer processes, frequency dispersion, as well as to homogeneities and roughness on the metal surface. Meanwhile, the diameter of the imperfect semicircles increases on increasing inhibitor concentrations. This observation suggests that the inhibitor strongly aggregates onto the aluminum surface, thus blocking the active sites and, therefore, increasing the charge transfer resistance, as well as the inhibition efficiency. The charge transfer resistance values (Rct) are calculated from the difference in impedance at lower and higher frequencies. The double-layer capacitance (Cdl) is obtained by the frequency at which the imaginary component of the impedance is maximum (-Zimax), and Cdl values are calculated from the following equation:
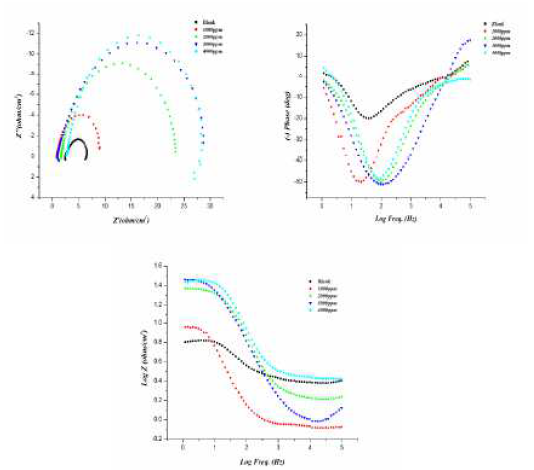
Figure 2 (a Nyquist plot for different concentrations of inhibitor. B) Bode plot (log f vs. log|Z|) and c) phase angle plot (log f vs. α0) for aluminum in 0.5 M HCl, without and with different concentrations of inhibitor.
The Bode plot, shown in Fig. 2© exhibited three different segments in the absence of inhibitor. In the high frequency region, the values of log |Z| and phase angle (α) tend to become zero, which is a characteristic response of a resistive behavior and corresponds to the solution resistance enclosed between the working electrode (aluminum) and the reference electrode (saturated calomel electrode). However, in the medium frequency region, there is a linear relationship between log|Z| against log f, with slope values close to -1, and the phase angle value tends to become -70, which is a characteristic response of a capacitive behavior. An ideal capacitor is associated with a slope value of -1 and a phase angle value of -90. The deviation from the ideal capacitive behavior in the present investigation is attributed to the rough working electrode surface, due to corrosion. The careful examination of the Bode plots reveals that the values of the phase angle significantly increased and therefore, the surface roughness remarkably decreased in the presence of inhibitor, due to the formation of a protective surface film. Further, Bode plots give one time constant, sigma maxima in the intermediate frequency region. The broadening of this maximum in the Bode plots is attributed to the adsorption and formation of a protective film by the L1 inhibitor at the metal/electrolyte interface.
Single depressed capacitive loops can be observed from the yquist plots (from Fig. 2(a), indicating charge transfer control during the corrosion process. With the increase in concentration of the inhibitor, the diameter of the loops increases, attaining its maximum value with the highest concentration of the inhibitor.
Galvanostatic polarization studies of the acid corrosion of aluminum in 0.5 M HCl, in the presence of Gymneme Sylvestre
The polarization curves of the aluminum electrode in 0.5 M HCl, in the presence and absence of the inhibitor, at various concentrations and a temperature of 298 K are shown in Fig. 3, and various electrochemical parameters are listed in Table 4.
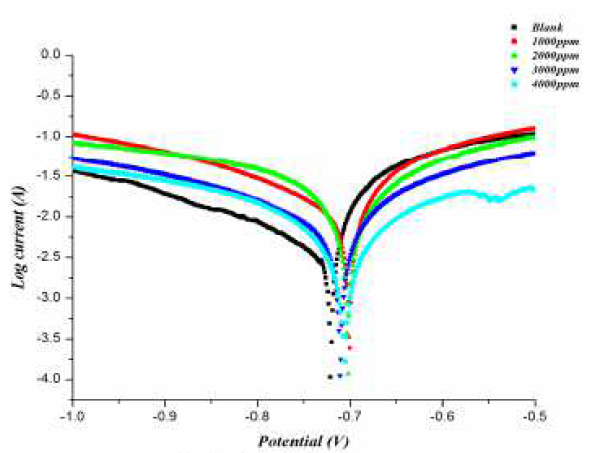
Figure 3 Polarization curves for aluminum, in the absence and presence of different concentrations of inhibitors.
It can be seen from polarization curves that the curves moved towards a lower current density region, in the presence of inhibitor. It can also be noted that both anodic and cathodic curves shifted towards lower current densities in the presence of inhibitor, without much change in Ecorr values. This finding suggests that investigated Gymneme Sylvestre inhibits aluminum corrosion in an acidic solution by forming a surface film without changing the mechanism of aluminum corrosion. Here, the inhibiting action can be explained by simple obstruction of the active site of the metal surface. In other words, the reduction of the corroded area is realized with covering of the surface by adsorbed organic molecules. Further, as the maximum change in Ecorr values is less than ±85 Mv, the investigated inhibitor can be considered as a mixed type inhibitor. The inhibitor shows a mixed type anodic behavior at 298 K, probably due to heteroatom electron density. The inhibition efficiency was obtained using the following expression:
Here, in the equation, Iacid is the corrosion current density of aluminum in an acidic medium only and Iadditive is the corrosion current density of aluminum in the presence of inhibitor.
Morphology
UV spectrum
The UV spectrum of water extract of Gymneme Sylvestre leaves as corrosion inhibitor of aluminum sheet, in presence of 0.5 M HCl, shows two peaks at 255nm and 365nm in Fig. 4.
The graph is showing a small red shift (bathochromic) in the presence of inhibitor with the aluminum strip, and also a small down shift which shows the absorption is intensity low (hypochromic) in the aluminum with inhibitor and in the blank inhibitor. The results obtained from the above spectrum indicate the formation of coordinate bonds between the inhibitor and the surface of the aluminum coupon.
IR Study
The water extract of inhibitor shows some broad IR peaks in Figs. 5.(a)- and (b).
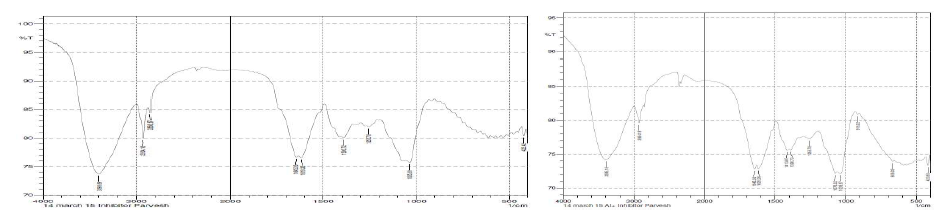
Figure 5 (a) IR spectra of pure inhibitor and (b) IR spectra of the inhibitor after interaction with aluminum.
In the blank inhibitor spectrum the peaks are: -OH peak at 3398 cm-1, =CH2(sp2) peak at 2924 cm-1, -CH3(sp3) peak at 2859 cm-1. -C=O has two peaks at 1645 cm-1; this is because the carbonyl present is hindered or not hindered by the other group present in our compound. The -C=C- stretching frequency was found out to be at a peak of 1618 cm-1. -C-O absorbance was found out to be at 1035 cm-1 and 1255 and 1390 cm-1 show the peaks of alcohol, ester group present in the inhibitor. The peaks at 1413 cm show the formation of an aromatic ring in the inhibitor. The absorption indicates the formation of an electrolyte on the inhibitor, which can be used as a protective layer.
Quantum chemical calculations
The computational methodology of quantum chemistry was being used in order to obtain the structure and electronic parameters. The value of EHOMO, ELUMO, ΔELUMO-HOMO and dipole moment are given in Tables 5-7.
Table 5 Calculated quantum chemical parameters of Gymnemic acid.
Quantum parameters | Gymnemic acid |
---|---|
HOMO( hartree ) | -0.17 |
LUMO( hartree ) | -0.06 |
ΔE LUMO-HOMO( hartree ) | 0.12 |
Dipole moment (μ) | 10.6 |
Table 6 Calculated quantum chemical parameters of Gymnestogenin.
Quantum parameters | Gymnestogenin | |
---|---|---|
HOMO ( artree ) | -0.12 | |
LUMO ( artree ) | 0.03 | |
ΔE LUMO-HOMO ( artree ) | 0.16 | |
Dipole moment (μ) | 4.7 |
Table 7 Calculated Quantum chemical parameters of Gymnemanol.
Quantum parameters | Gymnemanol |
---|---|
HOMO( hartree ) | -0.18 |
LUMO( hartree ) | 0.02 |
ΔE LUMO-HOMO( hartree ) | 0.21 |
Dipole moment (μ) | 5.20 |
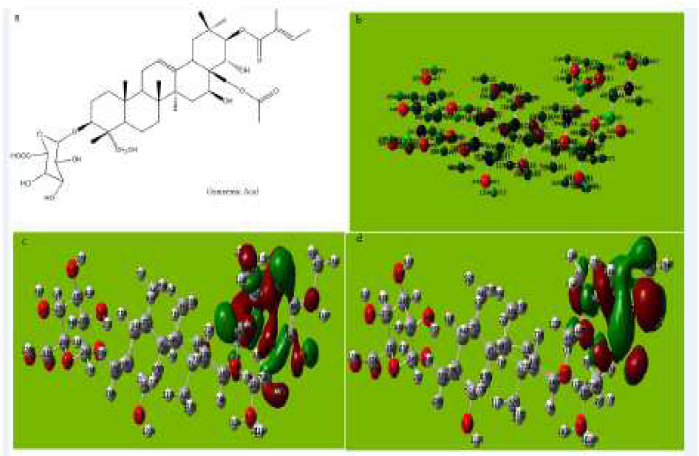
Figure 6 (a) Molecular structure with Mullikan charges of the Gymnemic acid. (b) Optimized molecular structure with Mullikan charges of Gymnemic acid. (c) HOMO of Gymnemic acid. (d) LUMO of Gymnemic acid.
The molecular structure of Gymnemic acid in Fig. 6a-e and Table 5 shows that the inhibitor gets adsorbed on aluminum by the formation of a coordinate bond by sharing of the electrons between oxygen atom and aluminum, hence giving rise to the π-electronic interaction.
The tendency of a molecule of Gymnemic acid to donate electrons to the acceptor molecule is shown by the value of EHOMO and ELUMO. The reactivity of Gymnemic acid towards aluminum is determined by the energy gap.
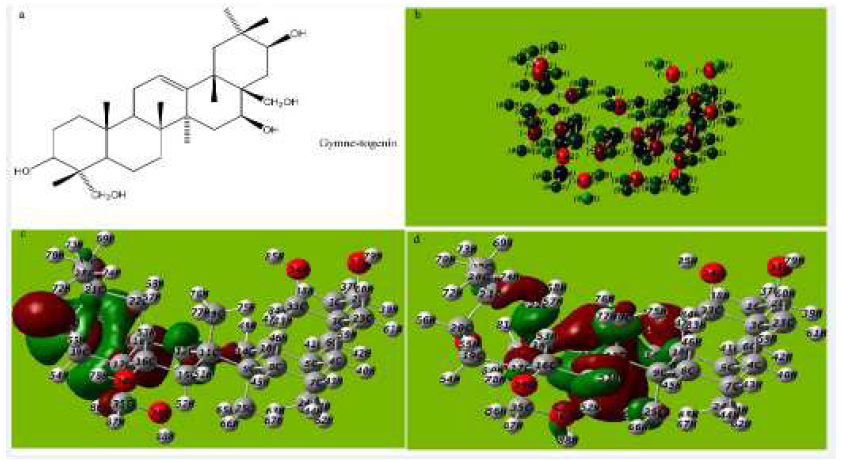
Figure 7 (a) Molecular structure with Mullikan charges of Gymnestogenin, (b) Optimized molecular structure with Mullikan charges of Gymnestogenin, (c) HOMO of Gymnestogenin, (d) LUMO of Gymnestogenin.
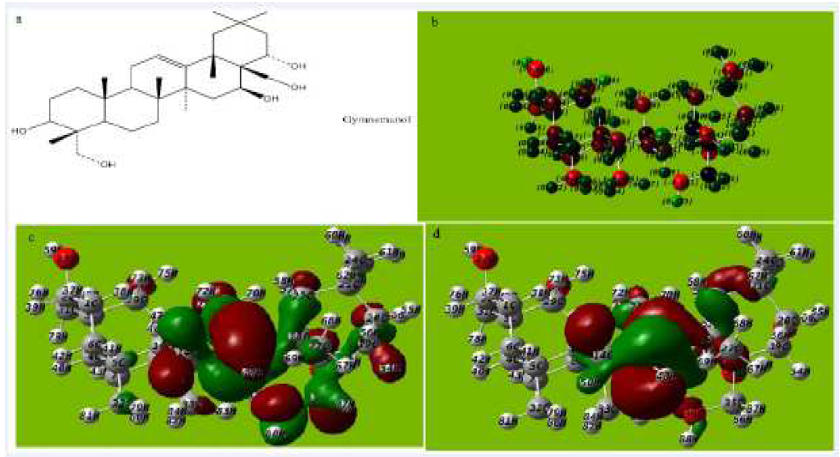
Figure 8 (a) Molecular structure with Mullikan charges of Gymnemanol, (b) Optimized molecular structure with Mullikan charges Gymnemanol, (c) HOMO of Gymnemanol, (d) LUMO of Gymnemanol.
The tendency of Gymnestogenin and Gymnemanol to donate electrons is given by the values of EHOMO, and to accept electrons is given by ELUMO, in Fig. 7a-e and Fig 8a-e and Tables 5, 6 and 7. The interaction of the inhibitor molecule with the surface of metal (aluminum) depends upon the electron transfer from the inhibitor to the aluminum surface.
Mechanism of adsorption and inhibition
The results show that the reason behind the adsorption at the metal/solution interface by the Gymneme Sylvestre leaves extract is the presence of free electrons on atoms such as oxygen and π bond between carbons present in Gymnemic acid, Gymnestogenin and Gymnemanol. As a result of these interactions, the formation of a complex takes place between aluminum and the inhibitor. The properties of inhibition are shown by the electron densities on the active site of the metal.
Conclusions
Corrosion inhibition properties of the inhibitor were investigated on aluminum in 0.5 M HCl using electrochemical methods, spectroscopic techniques, quantum chemical calculations. The following conclusions were drawn from the results:
Gymneme Sylvestre is a good inhibitor for the aluminum sheet at 0.5 M HCl. It shows maximum inhibition efficiency up to 82% at 800 ppm, and the efficiency of Gymneme Sylvestre on the aluminum sheet is seen to decrease with temperature variations and increases with increasing concentrations of the inhibitors.
Potentiodynamic polarization study showed that Gymneme Sylvestre is a mixed type inhibitor, and the EIS study revealed that Gymneme Sylvestre forms a protective film on the aluminum surface. The experimental results showed that the studied Gymneme Sylvestre adsorbs spontaneously onto the aluminum surface and conforms to the Langmuir adsorption isotherm.
FTIR spectra revealed the occurrence of chemical interactions between the inhibitor and aluminum.
Quantum chemical parameters such as EHOMO, ELUMO, and dipole moment agree with experimental results.