Introduction
Carbon steel is widely used in various industries, such as oil and gas exploration and production, petroleum refining, chemical manufacturing, water treatment, and in the product additive industries that play an important role in the economy 1,2. Acidic solutions employed in these industries lead to corrosion attack on the mild steel, which in turn generates a great loss 3. The use of organic inhibitors is one of the most practical methods for metals protection against corrosion. The organic molecules have the strongest adsorption ability on metal surfaces, which can markedly increase their inhibitory efficiency and change the corrosion resisting properties of metals 4,5. The inhibitory effect depends mainly on the nature and the surface charge of metals, on the chemical structure of organic molecules such as functional groups, steric factors, electron density, etc., and on the type of solution 6-9. The existing data in literature show that organic compounds having heteroatoms with high electron density, such as phosphorus, sulfur, nitrogen, oxygen or those containing multiple bonds, are effective inhibitors for metal corrosion 5-14. The objective of this study is the use of chemicals compounds with low or no environmental impacts at low dosages 15-17. On this content, we have recently focused on the inhibitory efficiencies of benzimidazole derivatives which are not toxic. 2-((5-Methyl-isoxazol-3-yl)-methyl)-benzimidazole (MMB) molecule contains many π- electrons, as well as one oxygen atom and three nitrogen atoms. The tested inhibitor has a molecular structure providing greater adsorption ability onto the carbon steel surface and high inhibitory efficiency. Due to the advantage of the organic compounds, which are described above, further detailed studies on the mechanism and stability of the inhibitor film on the metal surface would benefit contributions to its usage for practical applications. The aim of the present study is to investigate the adsorption and corrosion inhibition mechanism of MMB on carbon steel in a 1 M HCl solution. For this aim, potentiodynamic polarization and electrochemical impedance spectroscopy (EIS) techniques were used. The inhibitor molecule used in this paper has the structure presented in Fig. 1.
Experimental details
Inhibitor synthesis
Benzimidazole and its derivatives are an important class of bioactive molecules. We have redone the reaction of Azzaoui and al. 18, who have obtained the isoxazolyl-methylbenzimidazole1 from the action of hydroxylamine hydrochloride on 4Z-(2-oxopropylidene)-2,3,4,5-tetrahydro-1 H-1,5-benzodiazepin-2-one1 (Scheme 1).
To a solution of 4Z-(2-oxopropylidene)-1,2,4,5-tetrahydro-1H-1,5-benzodiazepin-2-one 1 (10 mmol) in ethanol, it was added hydroxylamine hydrochloride (10 mmol) and all was refluxed for a period of 2h. After neutralization with NaHCO3, the formed residue was recrystallized from ethyl acetate to afford the title compound 2 as colorless crystals. The compound is characterized by 1H-NMR (DMSO-d6): 2.36 (s,3H,CH3), 4.26 (s,2H, CH2), 6.23 (s,1H) and 7.00-7.60 (m,CHaromatique).
Preparation of electrodes and test solution
Corrosive solutions were prepared by dilution of a 37% HCl analytical reagent grade with doubly distilled water. The tested concentrations ranged from 10-3 to 10-6 M. Steel strips contained 0.36 wt.% C, 0.66 wt.% Mn, 0.27 wt.% Si, 0.02 wt.% S, 0.015 wt.% P, 0.21 wt.% Cr, 0.02 wt.% Mo, 0.22 wt.% Cu, 0.06 wt.% Al and the remainder iron.
The working area of 1 cm2 was subsequently ground with 200 and 1200 grit grinding papers, cleaned by distilled water and ethanol at hot air. The effect of temperature on the inhibition efficiencies of the inhibitor was tested from 303 to 333 K.
Electrochemical experiments
The effect of 2-((5-methyl-isoxazol-3-yl)-methyl)-benzimidazole (MMB) on the carbon steel corrosion was studied using electrochemical techniques: electrochemical impedance spectroscopy (EIS) and polarization potentiodynamic (PDP), in the concentration range of 10-3 to 10-6 M, at 303 K.
The electrochemical experiment consisted of a three electrode electrolytic cell made by a platinum foil as counter electrode, saturated calomel as reference electrode and carbon steel as working electrode, with an exposed area of 1 cm2.
The carbon steel specimen was immersed in a test solution for 30 min, until a steady-state (open circuit) potential was achieved using a type PGZ100 potentiostat. EIS measurements were performed with a frequency range from 100 KHz to 10 mHz and an amplitude of 10 mV with 10 points per decade.
The polarization curves were recorded by polarization from -600 to -200 mV/SCE, with a scan rate of 0.5 mV/s.
The data obtained by PDP and EIS methods were analyzed and fitted using graphing and analyzing impedance software, version EcLab.
Results and discussion
Effect of inhibitor concentration
Potentiodynamic polarization curves
Fig. 2 present the polarization curves for carbon steel in a 1 M HCl solution and in the presence of our compound tested at 303 K. Table 1 regroups the parameters derived from PDP plots. The values of ηPDP(%) are calculated by the following formula:
where and
are the corrosion current densities in uninhibited and inhibited media, respectively.
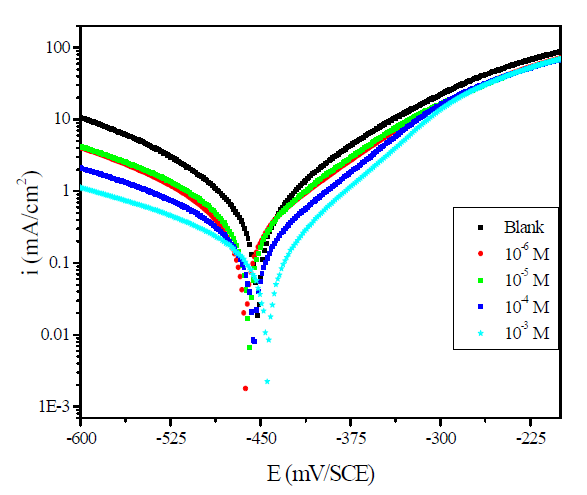
Figure 2 Potentiodynamic polarization curves for carbon steel in the absence and presence of different MMB concentrations.
Table 1 Tafel polarization parameters for carbon steel in a 1 M HCl solution, in the absence and at different concentrations of MMB at 303 K.
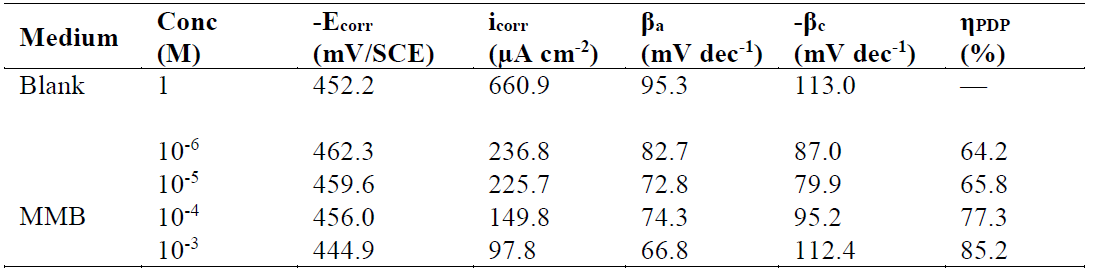
Analysis of the PDP curves indicates that the addition of MMB decreases both cathodic and anodic current densities with the increase in the inhibitor concentration. The presence of the inhibitor tested in the aggressive medium causes a slight change in the shift of Ecorr values in all tested concentrations, suggesting that MMB can be classified as a mixed type inhibitor 19, with no change in the metal corrosion mechanism 20.
Table 1 shows that the corrosion current densities (icorr) decrease when the MMB concentration rises in the acidic solution, reaching a value of 97.8 μA/cm2 at an inhibitor concentration of 10-3 M. This icorr value led to an ηPDP% of about 85.2 % and confirms that MMB is a good inhibitor against carbon steel corrosion in a HCl medium. A slight variation in the values of anodic and cathodic Tafel slopes is observed with the inhibitor presence. These results suggest that the surface blocking effect of the tested adsorbed compound diminishes the anodic and cathodic reactions 21.
Electrochemical impedance spectroscopy measurements
Impedance method provides information about the kinetics of the electrode processes and, simultaneously, about the surface properties of the investigated systems. So, the protective effectiveness of MMB on the carbon steel corrosion in the acidic medium (1 M HCl) was studied by EIS method at 303 K, after 30 min of immersion. Fig. 3 shows the influence of MMB concentrations on Nyquist impedance spectra.
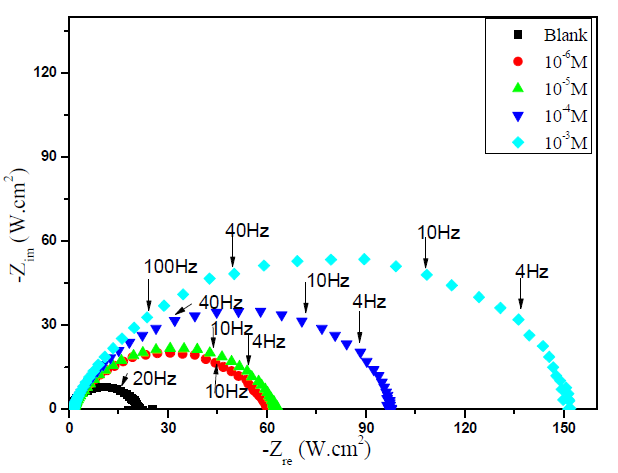
Figure 3 Nyquist plot for carbon steel in 1 M HCl, in the absence and presence of different concentrations of MMB at 303 K.
This figure shows that only single semicircles are observed with the depression at a low frequency, in MMB presence and absence. This depression is probably due to the inhomogeneity and roughness of the carbon steel surface 24. A remarkable increase in the semicircle diameter is observed when the concentration of MMB rises; this behavior is the result of the inhibitor adsorption onto the carbon steel surface 25. The equivalent circuit model shown in Fig. 4 was used to analyze the EIS experiments.Various impedance parameters, namely solution resistance (Rs), charge transfer resistance (Rct), double layer capacitance (Cdl), inhibition efficiency (ηz%) and surface coverage (θ) were calculated using the equivalent circuit model shown in Fig. 4 and given in Table 2.
Table 2 Electrochemical impedance spectroscopy parameters obtained for carbon steel in a 1 M HCl solution, in the absence and at different concentrations of MMB at 303 K.
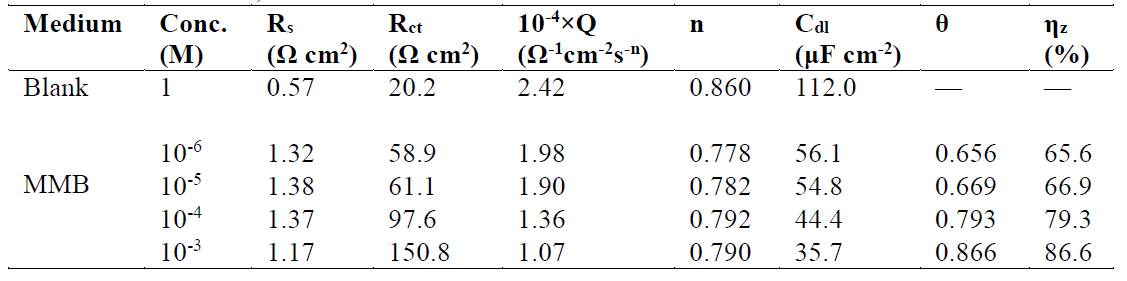
The inhibiting efficiency derived from EIS, ηZ (%), was calculated by the following equation:
where and
are the charge transfer resistance, in the inhibitor absence and presence, respectively.
The double layer capacitance (Cdl) values are calculated using Eq. (3):
where Q is the CPE constant and n is a coefficient that can be used as a measure of surface inhomogeneity 26.
From Table 2, it can be observed that Rct value increases with a higher concentration of MMB, while the Cdl values decreases. These results demonstrate that the compound was adsorbed onto the carbon steel surface by the formation of a protective film at the metal/solution interface 27-29.
The Cdl values diminished with the increase in the MMB concentration. The effect of the dielectric constant of a material on the interface capacitance can be related to the following equation:
where δ is the film thickness, ε the material dielectric constant and ε0 is the vacuum permittivity (8.8542 × 10-14 F cm-1). Based on the aforementioned, the increase in the MMB concentration reduces the interface dielectric effect. This behavior is due to a substitution process of adsorbed water molecules by MMB molecules, because their dielectric constants are lower than those of water. In this way, a protective film is formed, which diminishes the active sites on the steel surface and, consequently, the rate of the electrochemical corrosion reactions. The ηz% values obtained in the EIS study were in good agreement with those calculated in PDP studies.
Adsorption isotherms
The inhibition efficiency of the corrosion inhibitor mainly depends on the interaction between inhibitor and metal surface, and it can be provided by the adsorption isotherm 30. In order to evaluate MMB adsorption process in a carbon steel surface, Langmuir, Temkin, Freundlich and Frumkin adsorption isotherms were tested.
A plot of Cinh/θ versus Cinh (Fig. 5) was found to be the best fit, which suggests that MMB adsorption onto the carbon steel surface in the tested medium obeys the Langmuir adsorption. The Langmuir isotherm can be best expressed by the following relation (5):
where Cinh is the MMB concentration and Kads is the equilibrium constant for the adsorption−desorption process 31. From the intercept of the straight lines in Langmuir plot, the value of the Kads is calculated and given in Table 3. This value is also related to the standard free energy of adsorption, , by the following equation 6 32,33:
where the constant of 55.5 is the concentration of water in solution mol dm-3, R is the gas constant and T is the absolute temperature. The parameters of adsorption are regrouped in Table 3.
Table 3 Adsorption parameters for carbon steel corrosion in 1 M HCl at 303 K.
Inhibitor | Kads (M-1) | -ΔG° ads (KJ mol-1) | R2 | Slope |
---|---|---|---|---|
MMB | 1.323668×105 | 39.82 | 0.9997 | 1.1 |
The high Kads value obtained in this study indicates the strong interaction between the carbon steel surface and MMB adsorbing molecules 20. The calculated value of is around 39.82 kJ/mol, suggesting that the adsorption mechanism of MMB onto carbon steel in 1 M HCl is probably of a mixed type adsorption: physisorption and chemisorption 34.
Effect of temperature
The effect of temperature on the corrosion inhibition was studied using PDP measurement, in the temperature range from 303 to 333 K, without and with 10-3 M of MMB during 0.5 h of immersion. The electrochemical parameter extracted by PDP method, included in Table 4 and Fig. 6 (a and b), shows an increase in icorr with an increase in temperature, and it is more pronounced for the uninhibited solution. We observe that the inhibitor efficiency decreased with a rise in temperature, suggesting that the higher temperature does influence the inhibitor adsorption onto the metal surface.
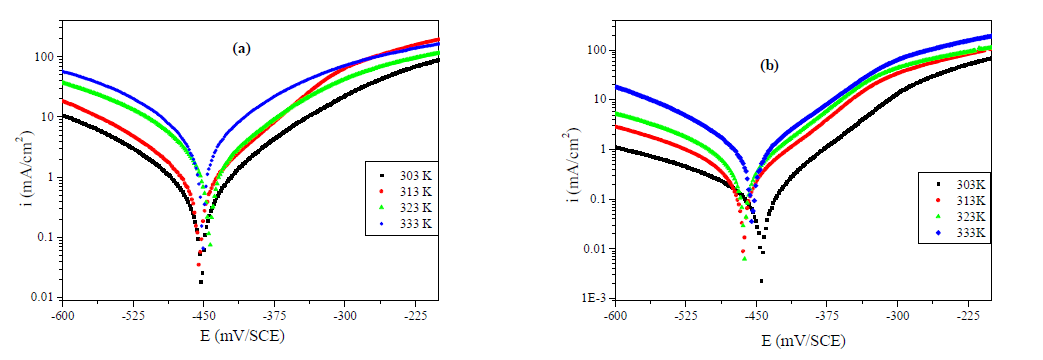
Figure 6 Effect of temperature on the behavior of carbon steel/ 1 M HCl interface in (a) uninhibited solution and (b) at 10-3M of MMB.
Table 4 Temperature influence on the PDP parameters for carbon steel in 1 M HCl, with and without HCl (1M) +10-3 M of MMB, at a temperature range from 303 K to 333 K.
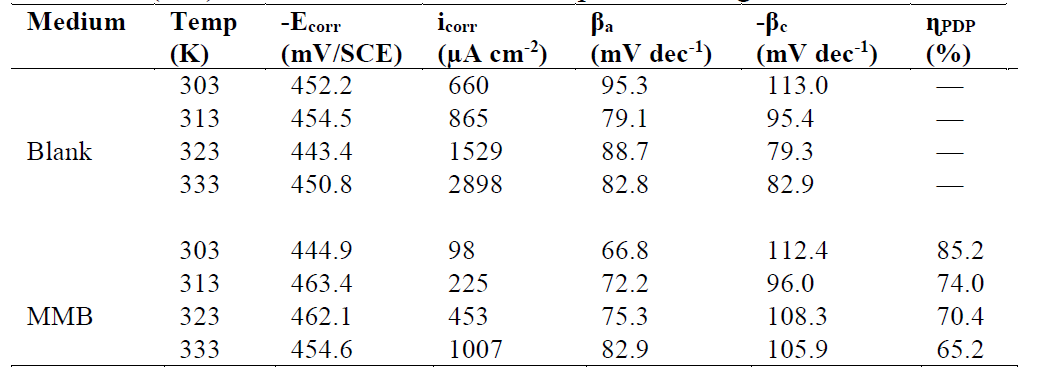
The effect of temperature on the corrosion current (icorr) can be used for determining the activation thermodynamic parameters for the dissolution process, following the Arrhenius equation (Eq.7) and transition state equation (Eq.8) (35,36):
where Ea is the activation corrosion energy, ∆Ha is the enthalpy, ∆Sa is the entropy of activation, T is the absolute temperature in Kelvin, h is the Plank constant, N is the Avogadro number and R is the molar gas constant. Arrhenius plots for the corrosion rate of carbon steel are given in Fig. 7. Using the slope of Ln (icorr) vs. 1/T plots, the values of the apparent activation energy of corrosion (Ea) have been determined (Table 5).
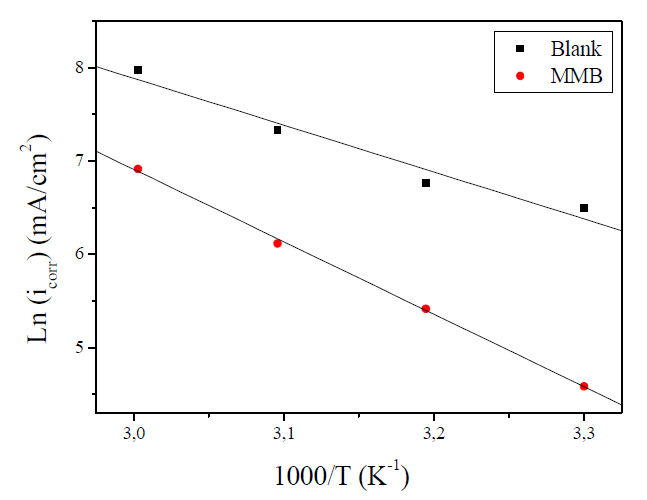
Figure 7 Arrhenius plot of Ln icorr versus 1000/T for carbon steel corrosion in 1 M HCl + 10-3 M of MMB.
The linear regression coefficient was close to 1, showing that carbon steel corrosion in HCl 1 M can be interpreted using the kinetic model. The Ea value calculated in an uninhibited solution (41.74 kJ mol-1) is in the same order of magnitude as previously described (13, 37). The obtained Ea value, in the presence of the tested compound, is 64.62 kJ mol-1.
Ea value, in the inhibitor presence, is more important than in its absence, indicating physical adsorption. The increase in activation energy explains the slight adsorption of the inhibitor onto the carbon steel surface with increasing temperatures 38,39. ∆Ha and ∆Sa values evaluated from the slope (∆Ha/R) and the intercept of (Ln(R/Nh) + (∆Sa /R)) of the plots of Ln(icorr/T) against 1/T (Fig. 8) are listed in Table 5.
The positive signs of ∆Ha reflect that the CS corrosion process is of an endothermic nature. The data of Table 5 show that ΔHa value is higher in the presence of the tested inhibitor (61.85 kJ mol-1) than that in its absence (41.24 kJ mol-1). ∆Sa value is more positive in a 1 M HCl solution containing MMB than that of the uninhibited solution. Some researches interpreted this behavior by the replacement process of water molecules during the adsorption of the inhibitor molecule onto the electrode surface 40,41.
Conclusion
2-((5-methyl-isoxazol-3-yl)-methyl)-benzimidazole constitutes an effective class of corrosion inhibitor for carbon steel in 1 M HCl. The efficiency increases with an increasing concentration, and maximum ɳ% was attained at 87% at 10-3M concentration. Polarization study revealed that the tested compound is a mixed type inhibitor, inhibiting cathodic and anodic reactions by forming a protective film onto the metal surface. MMB adsorption on the carbon steel surface obeys the Langmuir adsorption isotherm. The adsorption occurs by chemical and physical interactions. The results obtained from PDP and EIS are in good agreement.