Introduction
Phosphoric acid (H3PO4) is a medium-strong acid, but it still shows strong corrosiveness on ferrous and ferrous alloys 1; it is widely used in the production of fertilizers and steel surface treatment, such as chemical and electrolytic polishing or etching, chemical coloring, oxide film removal, phosphating, passivating and surface cleaning. There is a great need to protect steel materials used in the phosphoric acid industry. Up to now, little work 2), (3 appears to have been done on steel corrosion inhibition in H3PO4 solutions.
Considerable efforts are being made to find suitable compounds to be used as corrosion inhibitors in various corrosive media 4) - (12. One way to protect metal surfaces from oxidative corrosion is the use of the so-called corrosion inhibitors. Small amounts of these compounds are dissolved in corrosive media to protect the metal surface. To date, a large variety of different inhibitors has been studied in several corrosive media and on various metal surfaces 13) - (16.
Interestingly, there is a great interest in 1.4-benzothiazines which are increasingly being investigated for applications in the growing field of medicine. In fact, the 1,4-benzothiazines are the best known to possess biologically diverse activities 17 such as antimicrobial 18 antifungal 19 antioxidant agents 20 and anticancer 21), (22. Compounds based on the 1,4-benzothiazine moiety offer a high degree of structural diversity and have proven to be broadly and economically useful as therapeutics 23) - (25. It is the core structure in many drugs and biologically active natural products 26),(27. Sulfur-containing corrosion inhibitors have greatly interesting molecular structures compared with other classes of compounds. Their high protective effect for steels is due to the presence of the S atom in molecular structures 28. It is widely reported that highly efficient heteroatoms such as oxygen, nitrogen, phosphorus and sulfur follow the sequence of O < N < S < P, which makes sulfur-containing compounds excellent inhibitors 29), (30.
In the present work, mild steel (MS) corrosion inhibition effect in 2.0 M H3PO4 by Ethyl 3-hydroxy-2-(p-tolyl)-3,4-dihydro-2H-benzo[b][1,4]thiazine-3-carboxylate (EHBT) was investigated using weight loss, potentiodynamic polarization methods, electrochemical impedance spectroscopy (EIS) and scanning electron microscopy (SEM).
Experimental details
Synthesis
The general procedure for the preparation of two 1,4-benzothiazines derivatives was a solution of epoxide 1 (1 mmol) in acetonitrile (20 mL) added to 2-aminothiophenol (1 mmol). The mixture was refluxed for 25 h. The hydrogen cyanide was trapped by a 0.1 M KOH solution in a bubbler. Then, the solvent was removed under a reduced pressure, and the obtained residue was purified by flash chromatography on a silica column eluted with chloroform/ petroleum ether in the ratio of 2 : 1.
The result was Ethyl-3-hydroxy-2-(p-tolyl)-3,4-dihydro-2H-benzo[b][1,4]thiazine-3-carboxylate (EHBT): 0,19 g (60%). Infrared Spectroscopy (IR) for KBr (cm-1) gave the following results: 3378 (NH), 2982 (OH), 1746 (CO). The signals of diastereomer 1 were: 1H NMR (300 MHz, DMSO-d6): _ 2.40 (s, 3H, CH3), 4.7 (s, 1H, CHS), 1.28 (t, 3H, CO2CH2CH3), 4.7 (q, 2H, CO2CH2CH3), 6.4-7.5 (m, Ar); 13C NMR (75 MHz, DMSO-d6): _ 14.21, 21.49, 57.0, 63.92, 83.92, 115.28, 116.56, 122.69, 125.80, 127.22, 128.01, 130.36, 134.81, 140.14, 150.21 and 162.77. The signals of diastereomer 2 were: 1H NMR (300 MHz, DMSO-d6): _ 2.30 (s, 3H, CH3), 4.7 (s, 1H, CHS), 1.21 (t, 3H, CO2CH2CH3), 4.29 (q, 2H, CO2CH2CH3), 6.4-7.5 (m, Ar); 13C NMR (75 MHz, DMSOd6): _14.31, 21.34, 57.0, 62.37, 83.92, 114.29, 117.01, 123.17, 127.01, 127.59, 129.55, 130.75, 135.90, 141.92, 154.08 and 167.0. Electron Ionization Mass Spectroscopy (EIMS) results were: m/z (C18H19NO3S) 225 (100%), 116 (7.14%), 91 (7.14%) and 108 (17.14%).
Electrodes, chemicals and test solution
Corrosion tests have been performed, using gravimetric and electrochemical measurements, on electrodes cut from carbon steel sheets with the chemical composition: 0.370 % C, 0.230 % Si, 0.680 % Mn, 0.016 % S, 0.077 % Cr, 0.011 % Ti, 0.059 % Ni, 0.009 % Co, 0.160 % Cu and the remainder iron. The phosphoric acid aggressive media used for all studies were prepared by the dilution of analytical grade 85% H3PO4 with double distilled water. EHBT concentrations used in this investigation were varied from 10-4 to 5×10-3 M.
Gravimetric measurements
Gravimetric measurements were realized in a double walled glass cell equipped with a thermostat-cooling condenser. The used mild steel specimens with a rectangular form of the dimensions 2.5 × 2.0 × 0.2 cm3 were abraded with different grades of emery paper (320, 800 and 1200), and then washed thoroughly with distilled water and acetone. After accurately weighing, the specimens were immersed in beakers which contained 100 mL acid solutions, without and with various EHBT concentrations, at a temperature equal to 303 K, and remained immersed in a water thermostat for 6h. The gravimetric tests were performed by triplicate at the same conditions. The mild steel corrosion rates (CR) and inhibition efficiency (ηw %) have been evaluated from mass loss measurements using the following equations:
where CR and C' R are the mild steel corrosion rates in phosphoric acid, without and with the studied range of EHBT concentrations, respectively, and θ is the degree of surface coverage.
Electrochemical measurements
Electrochemical measurements, including stationary (PDP) and transient (EIS) methods were performed in a three-electrode cell. A pure mild steel specimen as working electrode, a saturated calomel (SCE) as reference electrode and an area platinum as counter electrode (CE) were used. All potentials were measured against SCE. The working electrode was immersed in a test solution for 30 min, until the corrosion potential of the equilibrium state (Ecorr) was achieved, using a type PGZ100 potentiostat. From Fig. 1, we can observe that there is no substantial variation in the open circuit potential for the last 15 min, confirming that the steady state was reached.
The potentiodynamic polarization curves were determined by a constant sweep rate of 1 mv/s. The transitory method (EIS) measurements were determined using ac amplitude signals of 10 mV peak to peak, at different conditions, in the frequency range from 100 kHz to 10 mHz. The data obtained by the EIS method were analyzed and fitted using graphing and analyzing impedance software, version Zview2. For the potentiodynamic polarization (PDP) method, the inhibition efficiency of the studied compound was calculated using the following equation:
where icorr and iº corr are the corrosion rates in the inhibitor presence and absence, respectively. The impedance diagrams were determined by the EIS method. To confirm reproductibility, all experiments were repeated three times, and the evaluated inaccuracy did not exceed 10%. For the EIS method, the inhibition efficiency was calculated using the following equation:
where Rp and Rp (inh) are the mild steel electrode polarisation resistance in the uninhibited and inhibited solutions, respectively.
In Fig. 2, OCP vs. time curves for mild steel in 2 M H3PO4, with the absence and presence of different EHBT concentrations, at 298 K, were recorded.
Results and discussion
Polarization results
In Fig. 3, the anodic and cathodic plots were recorded on the mild steel electrode in 2 M H3PO4, with the absence and presence of different EHBT concentrations, at 298 K. The associated electrochemical parameters, such as corrosion potential (Ecorr), corrosion current density (icorr), anodic and cathodic Tafel slopes (βa and βc), which were obtained from the intersection of anodic and cathodic Tafel lines, and the corrosion inhibition efficiencies, (PDP (0/0), were calculated, and are shown in Table 1.
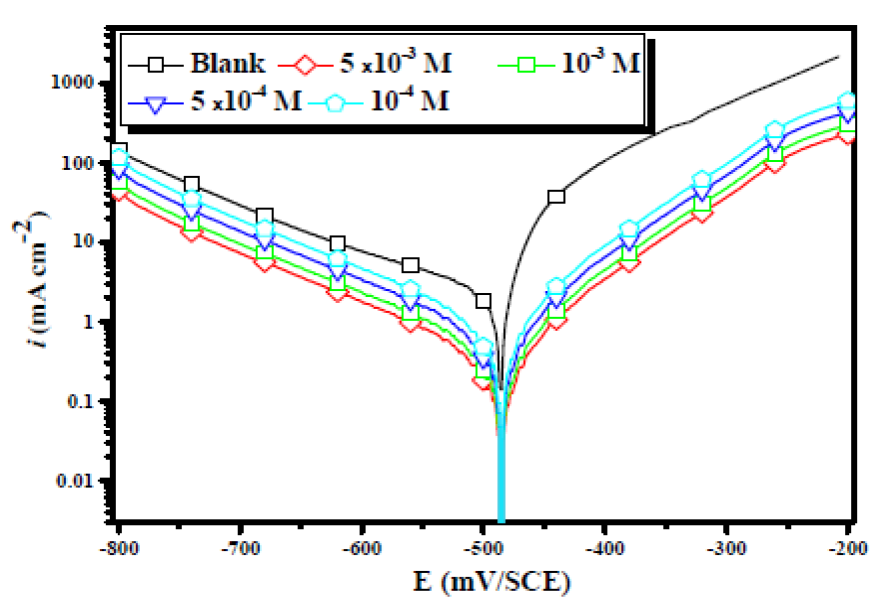
Figure 3 Potentiodynamic polarization curves for mild steel in a 2 M H3PO4 solution, in the presence and absence of different EHBT concentrations, at 298 K.
Table 1 Electrochemical parameters calculated by the PDP technique for mild steel corrosion in 2 M H3PO4, in the absence and presence of different EHBT concentrations, at 298 K.
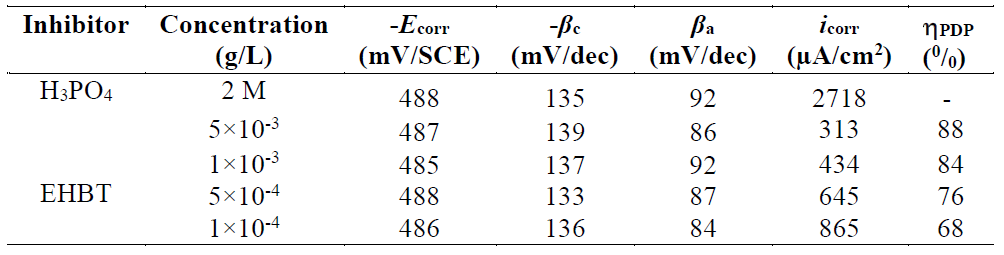
Moreover, all the cathodic curves were nearly parallel, suggesting that the cathodic reactions mechanism did not change in the inhibitor presence. This leads us to attribute the decreases in the cathodic current densities to the blockage of the cathodic sites caused by the EHBT molecules adsorpsion onto the mild steel surface, which has diminished the rates of both cathodic reactions. We have also concluded that the EHBT corrosion inhibition on mild steel was caused by a geometric blocking effect 31. Namely, the inhibition effect comes from the reduction of the reaction area on the corroding metal surface 31. βc and βa Tafel slopes did not change upon EHBT addition, which means that the inhibitor presence did not change the reaction mechanism. It can also be seen that both the anodic and cathodic currents were shifted to lower values, at the same potential, with higher inhibitor concentrations.
This behavior indicates that both the anodic and cathodic reactions were suppressed, i.e., EHBT is a mixed-type inhibitor 32),(33. In addition, it was observed that the higher the Cinh, the higher the (PDP (0/0), as a result of the increase in θ.
Electrochemical impedance spectroscopy (EIS)
Mild steel corrosive behavior, with and without the inhibitor in 2 M H3PO4, has been reported by employing EIS measurements, at 298 K. Nyquist plots on mild steel, in the absence and presence of various EHBT inhibitor concentrations in 2 M H3PO4, are given in Fig. 3. It was manifest that, with the EHBT addition to the H3PO4 aggressive solution, the mild steel impedance has significantly improved. The impedance plots for concentrations were found to be similar (Fig. 4).

Figure 4 Nyquist plots for carbon steel in a 2 M H3PO4 solution containing various EHBT concentrations, at 298 K.
Equivalent circuits are usually used to analyze EIS data 34) - (36. For example, Fig. 5 shows a simple equivalent circuit with one-time constant that is commonly used to analyze the measured impedance of a uniformly corroding electrode in an electrolyte.
This circuit consists of an electrolyte resistance (Rs), a polarization resistance (Rp) and a constant phase element (CPE), which is used for a non-ideal double layer. The non-ideal capacitance behavior of a double layer can be attributed to many factors, e.g., surface roughness and non-uniform surface coverage, corrosion rate or current distribution 37), (38. The impedance of this element is frequency-dependent, and it can be calculated using the following equation:
where Q is the CPE constant (in Ω-1 Sn cm-2), ω is the angular frequency (in rad s-1), j2 = -1 is the imaginary number and n is a CPE exponent which can be used as a gauge for the surface heterogeneity or roughness 39), (40. In addition, the double layer capacitances, Cdl, for a circuit including a CPE, were calculated by using the following equation:
It can be clearly seen from Fig. 4 that the Nyquist plots show a single capacitive semicircle shape at higher frequencies in the inhibitor presence. This indicates that mild steel corrosion in a 2 M H3PO4 solution is mainly controlled by a charge transfer process 41) - (43. The direct relationship between the capacitive loop diameter and the EHBT inhibitor concentration can be attributed to the increase in the polarization resistance (Rct), as a result of the increase in the corrosion inhibitor surface coverage 44. Consequently, the (EIS(0/0) value is expected to increase with higher inhibitor concentrations, exactly as is found and shown in Table 2.
Table 2 Impedance parameters recorded for the mild steel electrode in a 2 M H3PO4 solution, in the absence and presence of different inhibitor concentrations, at 298 K.
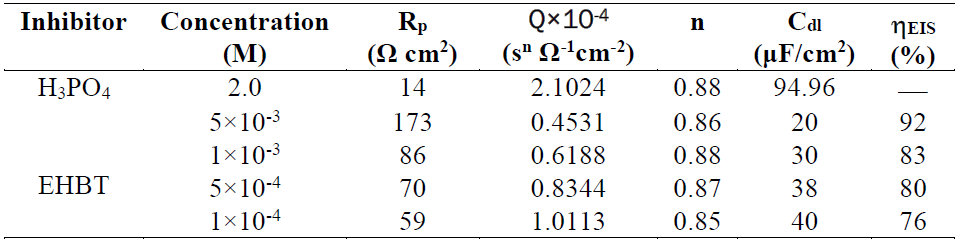
In Table 2, it is evident that the Rp values increase and that the capacitance values, Cdl, decrease with higher inhibitor concentrations. Decrease in Cdl, which can result from a reduction in the local dielectric constant and/or from an increase in the double layer thickness, suggests that the inhibitor molecules inhibit mild steel corrosion by an adsorption process at the metal-solution interface 45. It is well known that the capacitance is the reciprocal of the double layer thickness 46. A low capacitance value may also result in a larger replacement of water molecules by the inhibitor molecules, through the adsorption at the electrode surface 46. Also, larger molecules of the inhibitor may also reduce the capacitance through an increase in double layer capacitance. Moreover, the protective layer thickness that has increased with higher inhibitor concentrations results in a significant decrease in Cdl.
Weight loss study
The corrosion rate, inhibiting efficiencies and cover surface values obtained from the weight loss method, with different inhibitor concentrations, at 298 K, are given in Table 3.
It is clear from this table that the EHBT addition to the corrosive solution led to a decrease in the mild steel corrosion rate. Consequently, the inhibition effect and the surface coverage increased with higher concentrations of the tested inhibitor. The explanation of this behavior can be justified by the strong interaction of the inhibitor molecules with the metal surface, which results in adsorption.
Adsorption isotherm
Because the adsorption isotherm provides some structural and thermodynamic information, it is of great importance in the corrosion field 47) - (49. Generally, the inhibitors adsorption is largely influenced by the nature of the testing media, the chemical structure, the charge distribution, and by the metal nature 50), (51. To find a suitable adsorption isotherm in the present study, several commonly used isotherms were tested, among which the Langmuir adsorption isotherm was found to fit well with our experimental data (Fig. 6). The Langmuir isotherm can be represented as 52:
where Cinh is the concentration and Kads is the equilibrium constant of the adsorption process. Kads is related to the standard free energy of adsorption, ΔGº ads, by the following equation 52:
where R represents the gas constant and T is the absolute temperature. The value of 55.5 is the water concentration in the solution in mol/L.
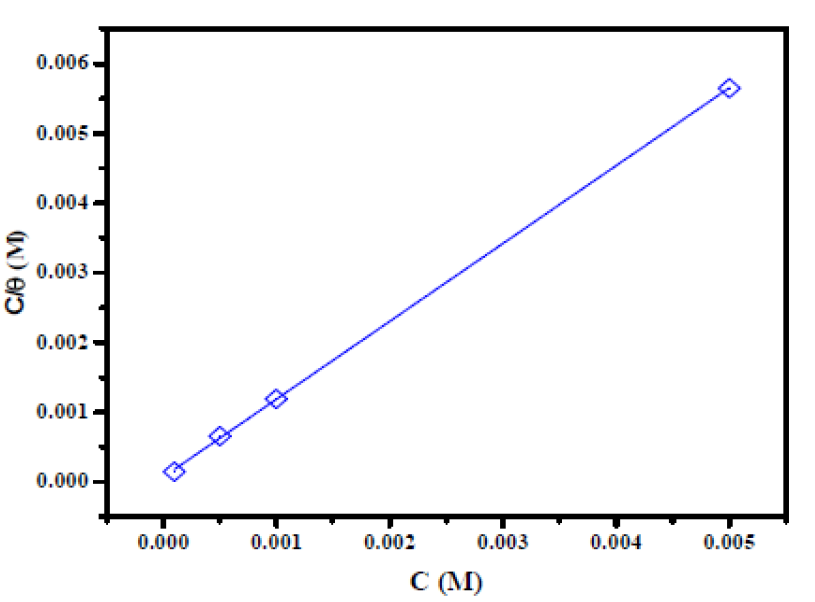
Figure 6 Langmuir adsorption of the inhibitor onto the mild steel surface in a 2 M H3PO4 solution, at 298K.
It has been mentioned in various literature that ΔGads values up to −20 kJ mol−1 are consistent with an electrostatic interaction between the charged inhibitor molecules and a charged metal (physical adsorption), while those nearly −40 kJ mol−1 or higher correspond to the charge sharing or charge transfer from the inhibitor molecules to the metal surface, to form a co-ordinate type of bond (chemical adsorption) 53),(54. The calculated ΔGads value for the studied inhibitor is -38 kJ mol−1 (Table 4). This suggests a chemical and physical mode of adsorption.
Surface characterization
SEM images of the mild steel surface were taken to analyze the morphology before and after the inhibition process. The MS sample, in the inhibitor absence, shows a rough and highly damaged surface, due to the rapid corrosion attack of 2.0 M H3PO4, as shown in Fig. 7 (b), compared to an un-attacked steel (Fig. 7(a)), while Fig. 7(c) shows a smooth and less damaged mild steel surface, in the inhibitor presence. The protected surface obtained for mild steel in the inhibitor presence might be attributed to the inhibitor molecules adsorption.
Conclusion
In the present work, a new benzothiazine derivative has been synthesized and then tested as a mild steel corrosion inhibitor in 2 M H3PO4. The tested compound (EHBT) acted as a good inhibitor for mild steel corrosion in a 2.0 M H3PO4 solution. Its inhibitive effect increased with higher concentrations, and slightly decreased at higher temperatures. EHBT acted as a mixed type inhibitor by blocking both cathodic and anodic corrosion reactions. EHBT adsorption onto the mild steel surface followed Langmuir adsorption isotherm. SEM images suggest that the inhibitor addition to aggressive solutions resulted in the formation of a protective film on the steel surface.