Introduction
MS is extensively used in oil and gas industries, but its inherent susceptibility to corrosive attacks in marine environments with high C of Cl- is a shortcoming to its applications in such pervasive media. Corrosion deteriorates MS properties, and can lead to its sudden failure 1. Marine environments affect steel pipelines integrity, according to corrosion experts in the field 2. Although many prevention strategies, such as anodic and cathodic protection, and the use of inhibitors and various deposition methods, have been applied to mitigate corrosion and its after effects 3, this phenomenon still occurs. The current trend has been challenging researchers to find alternative materials that will not only possess adequate AC properties, but that also meet the current global demand of environmental friendliness.
One of the important methods for mitigating corrosive attacks on steel constructions in numerous service environments is surface coating or modification. ED is one of such strategies used to fabricate coatings on metal surfaces in an electrochemical cell 4. This process enables the coated material to resist chemical attacks, and strengthens its capacity to withstand mechanical and physical loads. Besides, ED enables CD with low operational costs 5. CD involves the deposition of solid, liquid or gaseous adhering materials onto a substrate. The deposit can be a mixture of metallic and homogenous inactive materials, such as fibers and ceramic particles.
Metals like Zn, Ni and Al have been used as matrix materials in CD. For instance, microstructural, mechanical, wear and AC properties of Zn-based CC have been enhanced using non-metal additives such as particles of carbides, metal oxides, nitrides, borides and silicates, e.g. TiO26,7, SiO28,9, Al2O310 and TiB211. Other additives include graphite particles 12, silver nanoparticles and polymers. CC made of inorganic and organic additives have proved to be effective in AC.
Specifically, Zn CC embedded with tin oxide elements prepared by 13, through ED from a sulphate bath, displayed enhanced AC properties in a NaCl solution, due to the embedded particles. Popoola et al. 14 also performed Zn and Al powder ED on MS substrates, in order to form CC. The results showed that the microhardness of the coated samples was enhanced up to 92%, while CR decreased by 90%, and AC properties improved. 15 prepared Zn CC embedded with MoS2 nanoparticles from a ZnS-Cl-1 bath, and the morphological and AC properties were analyzed. The corrosion behavior and microstructure assessed by PDP and SEM/EDXS technique, respectively, indicated improved AC and morphological properties.
Corrosion mitigation using CD is an easy and effective AC method, but most CC are expensive, toxic and non-eco-friendly. Therefore, researchers have been searching for AC materials that are cheaper and non-toxic. A wide spectrum of readily available, inexpensive and non-toxic additives or particulates has been studied. For instance, 16 explored Zn-ZnO ED with the addition of anthill sand particles and orange juice as surfactants. The (, CR and AC properties were significantly enhanced, with corrosion resistance values of 94.41 and 92.86% for the coating with and without orange juice, respectively. 17 also performed Zn-ZnO CD with snail shell particles on MS, and studied its effect on the microstructure, (, CR and AC properties of the coatings in simulated SW. The results proved that MS coating with snail shell particles improved ( and AC properties, and decreased CR. 18 also carried out Zn-ZnO CD with RH ash from a Cl-based bath, and found out that RH additives improved MS AC properties in a 3.5% NaCl solution. Another study found out that RH improved both ( and CR 19. Following 16 studies, who performed Zn-ZnO CD with anthill particles, 17, who carried out Zn-ZnO CD with animal waste, and 18,19, who used RH ash additive, the present study explored DLCFA and LSLA AC effect.
LC fruit is normally found in subtropical regions and in various locations in Nigeria. This plant belongs to the Cucurbitacea family, and it has a high fiber content of 54.2% and 15.10% cellulose and lignin, respectively 20. Lamiaceae plant is known for its broad leaves, being also found in Nigeria, and it contains high Fe, Cu, Zn and Mn contents 21. SS content is high in quartz, being abundant in Nigeria, but is mainly used for roads and building construction, thus leaving its huge deposit under-utilized 22. This work has assessed the AC effect of Zn-ZnO ED, with natural and eco-friendly additives made of two plant wastes (DLCFA and LSLA) and SS, on MS corrosion in simulated SW. The study outcome revealed the potential of incorporating DLCFA, LSLA plant waste additives and SS particulates into Zn-ZnO, as useful AC materials.
Materials and methods
Materials
Materials used for this work included DLCFA and LSLA AW, SS, a MS sheet and chemicals for the ED bath. LC fruits shown in Fig. 1 were collected from a farm near the University of Nigeria in Nsukka, Enugu state.
LC samples were deseeded, crushed and carbonized in a carbolite furnace, at 700 ºC, for 2 h, and allowed to cool inside it. The carbonized fruits (ash) were later sieved. of Lamiacea shed leaves shown in Fig. 2 were also collected from University of Nigeria botanical garden, and treated just like DLCFA.
SS was collected at Akwuke beach, along Enugu-Port Harcourt highway, in Enugu state, Nigeria. SS samples were dried for six weeks, pulverized and sieved, for obtaining particulate sizes of 75 μm. Sieved SS is shown in Fig. 3.
MS was supplied by Auskan Co. Ltd, Kaduna State, Nigeria. It was cut into a sheet with the dimensions of 20 x 20 x 4 mm, ground and polished with 200-1200 grit SiC papers, followed by cleaning with ethanol, drying and storage. The polished MS samples were numbered, and used as substrates for ED. The deposition bath compounding chemicals were purchased from JOECHEM Chemical store, Nsukka, Nigeria. They were similar to the compounds used by 16 in their work, but C were varied in the present study. The chemicals were ZnCl, NH4Cl, H3BO3, thiourea and ZnO.
Methods
Determination of MS elemental composition
MS elemental composition was determined using Spark model Spectro Maxx arc/spark OES metal analyzers.
Deposition process
ED was prepared using a bath composition similar to that 16, but both bath and additives C were varied, as shown in Table 1. All chemicals used to prepare the electrolytic bath (Table 1) were in powder and solid forms. ZnCl2 powder (100 g/L) was dissolved in water, in order to form an acid solution, and obtain Zn2+ and HClaq. The acid solution aided in masking H from preferential discharge. 15 g/L ZnO were also added to the water. Although ZnO is not wholly soluble in water, it acted as one of the particles that were deposited on the cathode. Zn powder (15 g/L) was used to replenish Zn2+ depletion during plating. NH4Cl salt (15 g/L) was equally added to the solution. By injecting HClaq, NH4Cl dissolution helped maintaining the solution acidic nature. During ED, H3BO3 (15 g/L) enabled DLCFA, LSLA and SS particles inert circulation in the bath. Thiourea acted as organic surfactant. Thus, the electrolytic bath was an aqueous suspension of ZnCl2, H2O, ZnO, H3BO3, Zn, NH4Cl and thiourea, being prepared before CD. The sample codes and deposition design are shown in Table 2.
Table 1: Electrolytic bath composition.
Composition | Chemical phase | C (g/L) |
---|---|---|
ZnCl | Powder (s) | 100 |
NH4Cl | Powder (s) | 15 |
H3BO3 | Powder (s) | 15 |
Thiourea | Powder (s) | 15 |
ZnO2 | Powder (s) | 15 |
Additives | ||
DLCFA | Powder (s) | 0, 5, 15, 25 |
LSLA | Powder (s) | 0, 5, 15, 25 |
SS | Powder (s) | 0, 5, 15, 25 |
Sample code | Coating composition |
---|---|
A (control) | MS sample coated with Zn-ZnO without additives |
B | MS sample coated with Zn-ZnO + 5 g/L DCLFA |
C | MS sample coated with Zn-ZnO + 5 g/L LSLA |
D | MS sample coated with Zn-ZnO + 5 g/L SS |
H | MS sample coated with Zn-ZnO + 15 g/L DCLFA |
I | MS sample coated with Zn-ZnO + 15 g/L LSLA |
J | MS sample coated with Zn-ZnO + 15 g/L SS |
N | MS sample coated with Zn-ZnO + 25 g/L DCLFA |
O | MS sample coated with Zn-ZnO + 25 g/L L LSLA |
P | MS sample coated with Zn-ZnO + 25 g/L SS |
Each CD process was performed using a direct I rectifier, which was operated at a voltage of 1.5 V and I of 0.6 A, during ED. ED set up is shown in Fig. 4.
The polished MS sample, which served as cathode, was connected to the rectifier black terminal, and Zn anode was connected to the red terminal. ED parameters were: time = 30 min, T = 65 ºC, and solution stirring rate = 400 rpm. The coating formulations were: Zn-ZnO-x DCLFA particulates (x = 0, 5, 15 and 25 g/L); Zn-ZnO-y LSLA (y = 0, 5, 15 and 25 g/L); and Zn-ZnO-z SS particles (z = 0, 5, 15 and 25 g/L). After ED, the coated samples were rinsed in distilled water, dried and stored for characterization.
Corrosion test
The corrosion behavior of both coated and uncoated samples was determined in simulated SW, using PDP. The test was carried out using a three electrode cell potentiostat. During each test, the coated sample served as WE, while a SCE and a graphite electrode functioned as RE and CE, respectively. The three electrode measurement system was an electrochemical analyzer (CH Instruments, Model CHI604E, USA). Each test was performed in 250 mL SW, with an applied E in the range from -0.5 to +0.5 V (vs. SCE), at a SR of 0.01 V/s.
Microhardness test
The coated samples ( was assessed using a Leeb micro-hardness testing machine (Model: PRLH210) shown in Fig. 5. Three indentations were done on the surface of the samples, and average ( was recorded.
Microstructural analysis
The microstructures of the coated samples were analyzed using a Mode VEGA 3 TESCAN SEM coupled with EDXS.
Results and discussion
MS elemental composition
MS elemental composition is shown in Table 3. The carbon content was very much below the upper limit of low CS (0.25 wt.% C).
Table 3: MS elemental composition.
Element | C | Si | Mn | P | Cr | Al | Cu | Fe |
---|---|---|---|---|---|---|---|---|
Composition (wt. %) | 0.15 | 0.01 | 0.32 | 0.02 | 0.04 | 0.01 | 0.01 | 99.44 |
Microstructural analysis
Figures 6, 7, 8 and 9 show the scanned images of some of the coated MS samples containing low and high C of additives, at 100 μm magnification. Fig. 6 shows the MS sample A (coated with Zn-ZnO without additives) microstructure. Figs. 7,8 and 9 are the micrographs of MS samples coated with: Zn-ZnO + 5 g/L DLCFA (B); Zn-ZnO + 15 g/L LSLA (I); and Zn-ZnO + 25 g/L SS (P). There were some morphological differences among the coated MS samples.
Result of ( tests
The variation in average ( of the uncoated and coated samples with various additives C is shown in Fig. 10.
Fig. 10 indicates that the average ( of the coated samples increased after ED. DLCFA coated samples ( increased from 90 HB to 94 HB, as their particulates C were risen from 0 to 25 g/L. Similar improvement in ( with an increase in C was observed for LSLA and SS coated samples. LSLA samples ( did not increase as much as that of DLCFA or SS coated samples. This implies that LSLA samples are the softest. However, SS coated samples displayed high ( values of up to 97 HB. Similar results were also observed by 16, who performed Zn-ZnO CD with anthill sand particles, where the increase in ( was attributed to their hard and abrasive nature.
Corrosion characteristics of the coated and uncoated MS samples
Figs. 11, 12 and 13 show polarization curves of uncoated MS samples and of those coated with Zn-ZnO only, and with varying additives C.
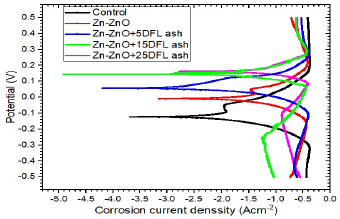
Figure 11: E vs I for uncoated MS samples and those coated with Zn-ZnO only and with varying C of DLCFA particulates.
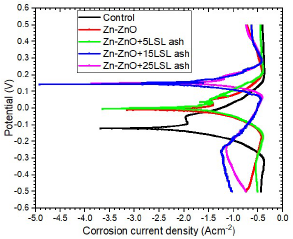
Figure 12: E vs I for uncoated MS samples and those coated with Zn-ZnO only and with varying C of LSLA particulates.
From Figs. 11, 12 and 13, it can be seen that MS coating with Zn-ZnO only (without additives) and with additives had Ecorr more positive values, which caused a decrease in icorr and CR values of the coated steel substrates in SW, as shown in Table 4. The coated samples AC abilities also increased with higher additives C. MS coated Zn-by ZnO without additives equally displayed higher corrosion resistance than the uncoated MS sample. However, the SS coating offered most protection to the steel samples, followed by the DLCFA and, lastly, by LSLA particulates.
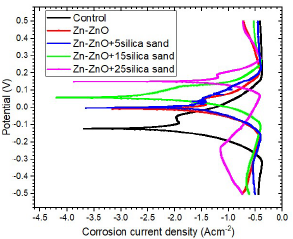
Figure 13: Potential vs. I for uncoated MS and samples coated with Zn-ZnO only and with varying C of SS particulates.
SS additive was the only one with a significant effect on the substrates CR reduction. The highest corrosion resistance was seen for the substrate coated with SS additive, at 25 g/L C, which had the lowest CR of 6.13 × 102 mm/yr (61.5% improvement), followed by that coated with DLCFA (53.8% improvement) and by that coated with LSLA particulates (44.3% improvement).
Table 4: Corrosion data obtained from PDP for uncoated and coated MS samples.
Sample code | Composition | PDP test data | ||
---|---|---|---|---|
Ecorr (V) | icorr (A) | CR (mm/yr) | ||
Control | Uncoated | -0.12 | 1.05 E -02 | 1.59 E +03 |
A | Coated with Zn-ZnO without additives | -0.02 | 1.03 E -02 | 1.55 E +03 |
B | Coated with Zn-ZnO + 5 g/L DLCFA | 0.08 | 9.74 E -03 | 1.15 E +03 |
H | Coated with Zn-ZnO + 15 g/ L DLCFA | 0.15 | 8.82 E -03 | 9.80 E +02 |
N | Coated with Zn-ZnO + 25 g/ L DLF ash | 0.18 | 5.84 E -03 | 7.35 E +02 |
C | Coated with Zn-ZnO + 5 g/ L LSLA | 0.02 | 6.42 E -03 | 1.12 E +03 |
I | Coated with Zn-ZnO + 15 g/ L LSLA | 0.14 | 5.96 E-03 | 9.95 E +02 |
O | Coated with Zn-ZnO + 25 g/ L LSLA | 0.16 | 3.29 E -03 | 8.85 E +02 |
D | Coated with Zn-ZnO + 5 g/ L SS | 0.00 | 8.92 E -03 | 1.55 E +03 |
J | Coated with Zn-ZnO + 15 g/ L SS | 0.06 | 7.82 E -03 | 9.65 E +02 |
P | Coated with Zn-ZnO + 25 g/ L SS | 0.16 | 6.06 E -03 | 6.13 E +02 |
The high protection efficiency by SS might be due to Si presence, a ceramic material that has been found to impede steel dissolution in SW 23, or to the formation of more passive and protective films on the coated MS surfaces, due to the deposited particles reaction with SW. Thus, SS fulfilled one of the necessary requirements for CR reduction, which is to generically decrease icorr or increase Ecorr. Similar observation was also made by 16, who performed Zn-ZnO CD with anthill sand particles, which increased AC properties significantly. LSLA particulates lower CP was partly due to their σ, which caused their easy erosion in SW.
Conclusion
In this work, ED of a Zn-ZnO composite separately incorporating DLCFA, LSLA and SS particles as additives, was carried out on MS substrates. The coatings effects on AC properties, microstructural evolution and ( of MS coated samples in SW were assessed, and the results are as follows:
There was an increase in the coated MS samples ( values, particularly in the SS coating samples. The sample coated with 25 g/L SS particulates exhibited the highest (.
MS coating improved its AC properties. However, the composite coating that incorporated SS particles displayed the highest AC properties in SW. Coating with DLCFA and LSLA additives also improved MS AC properties, but their CP were below that of SS particles.
LSLA particles had the lowest CP for MS in a SW environment, and this might be due to their low (, which made the coated samples very prone to erosion, due to low resistance offered by the soft surfaces.
Authors’ contributions
C. C. Daniel-Mkpume: conceived the research idea and supervised work. F. S. Ugwoke: collected samples, prepared solutions and performed the experiments. K. N. Mkpume: inserted data or analysis tools. E. G. Okonkwo: designed and performed the analysis. C. Ocheri: designed and set up the deposit platform. P. O. Offor: contributed to the paper writing. A. D. Omah: verified the numerical results. C. S. Obayi: wrote the manuscript in consultation with all authors.
Abbreviations
AC: anticorrosion
AW: agro-waste
C: concentration
CS: carbon steel
CD: co-deposition
CC: composite coatings
CE: counter electrode
Cl-: chloride ion
CP: corrosion protection
CR: corrosion rate
DLCFA: dry Luffa cylindrica fruit ash
E: potential
Ecorr: corrosion potential
ED: electrodeposition/electrodeposited
EDXS: energy-dispersive X-ray spectroscopy
icorr: corrosion current
IE(%): inhibition efficiency
H3BO3: boric acid
LC: Luffa cylindrica
LSLA: Lamiaceae (teak) shed leaves ash
MS: mild steel
NaCl: sodium chloride
NH4Cl: ammonium chloride
OES: optical emission spectrometry
PDP: potentiodynamic polarization
RE: reference electrode
RH: rice husk
SCE: saturated calomel electrode
SEM: scanning electron microscopy
SiC: silicon carbide
SR: scanning rate
SS: silica sand
SW: sea water
T: temperature
WE: working electrode