Introduction
ISE is a transducer or sensor comprises a polymeric host membrane and sensing components which converts the specific ion activities or ion Ct in solution into an electrical potential. The polymeric membrane plays an essential role in the construction of ISE 1-4. In general, the membrane has two main roles. Firstly, the membrane acts as a supporting matrix capable to retain its sensing components such as liphophilic salts, ionophores, and plasticizers which functions to reduce the glass transition properties (Tg) of the membrane 5. Secondly, the membrane also acts as a separator matrix in between the aqueous sample and the surface of ISE transducer or internal layer 1,6-7. Fig. 1 shows the structural construction for cationic ISE.
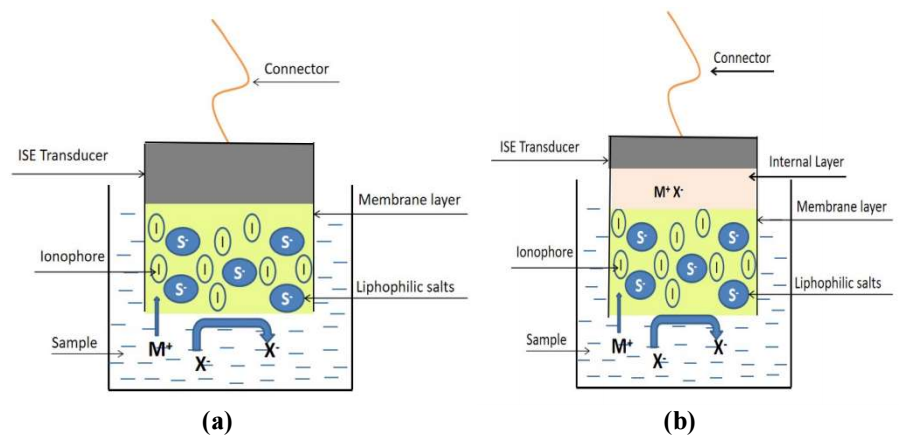
Figure 1: Illustration of an ISE sensor structure (a) without internal layer, (b) with internal layer
In ISE development, there are several important characteristics the membrane, prior to the sensor fabrication process. The polymeric membrane used in ISE must have stable properties, so that it does not easily dissolve in the sample or undergo a redox reaction. Furthermore, the membrane must possess low or non-toxic properties, considering that some of the samples used are medical samples whereby measurements are conducted invasively and used for the blood vessels. In addition, the membrane also must be able to work well with all the sensor components needed for fabrication of ISE sensors such as lipophilic salts, ionophores, plasticizers and also organic solvents 6-7. On top of that, the polymeric glass transition as low as -40 - 15 ºC is preferred, to allow ion transfer from the sample onto the membrane to occur properly and the sensing components inside the membrane would not easily leach-off 2,6-8. Hence the challenge to produce a desirable polymeric host matrix compatible for detection and measurement of lead (II) ions for Pb-ISE is great of interest 3.
Pb (II) ion is one of the heavy metal ions which is very dangerous for the environment, living things and humans. This metal is very toxic and it can bring serious problems to the environment and even disrupt human health through in trace amounts exposure. According to the WHO, the maximum traceability limit of Pb (II) ion in drinking water is 10 g/L even lower at 7.2 g/L2 as recommended by European WFD 2,9-10. The accumulation of lead (II) ion in human can cause serious damage to human organs such as liver, bone, nervous system, brain, and other important organs in humans, which in turn can cause death 11. Typically lead (II) ion exposure to humans occurs through food chain systems, air pollution, and workplace environmental conditions 12-13.
Conventionally, PVC type of membrane is commonly used in the fabrication of Pb-ISE sensors 14-18. However, this type of membrane has high Tg value, which requires addition of plasticizers to reduce its Tg value and, consequently, helps to increase the segmental motion of the polymer backbone and generate free volume for mobility of sensing components in polymer structure 2,6,8. Adversely, the plasticized type membrane is not recommended for medical applications, especially for invasive type ISE sensor in blood vessels. This is mainly because plasticizers are generally toxic, that when plasticizers are leaching, it certainly will poison the blood 6,19. In addition, the main disadvantage of PVC-ISE fabrication is that it requires long and complicated process. PVC membranes normally have longer evaporation time for the drying process to form thin films. Generally, the evaporation process takes about 12-24 h. Another disadvantage of PVC membrane is it has poor adhesive on the surface of the electrode, which causes the PVC membrane to easily peel off from electrode surface. To overcome this issue, a membrane clamping technique is usually done. However, due to small size of ISE electrode or SPE shape, it certainly leads to a complex sensor fabrication and design 2,6,8.
The limitations shown by the PVC type membrane in the fabrication of Pb-ISE sensors have encouraged researchers to continue to develop membranes which is suitable for producing Pb-ISE sensors. Another polymer host membrane developed for ISE is using PHXA as an alternative to PVC membranes 20. The advantage of PHXA membrane is it is able to function as sensing membrane even though without addition of plasticizers. However, this membrane is still unfavourable due to its poor selectivity and complicated fabrication process which takes up 1 week of drying time.
Another alternative sensing membrane is based on polyacrylate. This type of membrane has several advantages over previous reported membranes, including being able to function without using plasticizers, the available monomers vary that their mechanical properties can be adjusted, has good adhesive on the surface and it can be prepared either through thermo-polymerization or through the PP process 3,21. The development of the Pb- ISE based on polyacrylate has been reported by previous researchers such as 22, using pIDA. The pIDA membrane can function well as a Pb-ISE sensor even without the presence of plasticizers across the material. Such approach, however, has a fundamental drawback such as the preparation process involving a thermo-polymerization process that requires a lot of solvents, longer heating time and drying time that reaches 24h. Multiple steps and longer times are needed for the fabrication process 22. The same unfavourable fabrication process was carried out by 23 using MB from CP thin films membrane.
The long preparation time will certainly lead to higher Pb-ISE fabrication costs. So, another alternative method to produce a rapid polymeric host membrane is using the PP technique within a few minutes 2,8,24. Pb-ISE sensor developed based on polyacrylate PP has been examined by 24, using pBA and CP from pBA-HEMA. Both of these Pb-ISEs show very good sensor responses, especially sensor which has been fabricated with pBA-HEMA CP. However, the developed pBA and pBA-HEMA based membranes are unfavourably very soft and have slightly sticky properties 2,8. Mishandling of the membrane will cause a torn off membrane, and pBA-HEMA is likely to stick on the beaker glass walls during measurement.
Another alternative is to use pTHFA PP membrane developed by 2. This Pb-ISE sensor has shown better mechanical properties than those of pBA, but the sensor linear measurement range is only up to 10-5 M. Thus, the performance of the Pb-ISE sensor can still be improved. In this study we have investigated the effect of adding HEMA monomer as lead (II) sensing membrane. HEMA membrane is a hydrophilic acrylate membrane which is commonly applied as an ISE sensor inner layer. Typically, the pHEMA membranes are often used as supporting matrices in immobilizing biological materials for bio-sensor applications. The excellent property of hydrophilic pHEMA has helped to promote the ion transports from the sample to the surface of the electrode or transducer 25-26.
Based on the above description, thus in this study, the development of the Pb-ISE fabrication process is carried out using the PP technique, wherein the CP of the pTHFA-co-HEMA membrane is used as a material for fabrication. The addition of HEMA monomers is expected to improve the performance of the Pb-ISE based pTHFA. This research includes testing the structure and also the Tg value of p(THFA-co-HEMA) by using FTIR, and DSC. Besides that, the performance measurement of Pb-ISE sensors includes testing on linear range, LOD, selectivity, pH effect and finally validation testing using AAS method is carried out using both artificial solutions and real samples.
Methodology
Instrumentations
The equipment used in this study includes the Corrtest CS350 potentiostat, platinum electrodes (Pt) and standard calomel electrodes (SCE) found from Wuhan Corr Test Instrument Corp. Ltd., 9V battery, Ag-SPE from Scrint Print, Sdn Bhd-Malaysia, UV-box exposure from Huanyu Instrument, TGA/DSC (DTA PT1600 Linsesis), and FTIR (Thermoscientific Nicolet iS-10).
Materials
The chemicals used in this study are monomers THFA, HEMA purchased from Merck. Lead Ionophore (IV), HDDA as a cross-linker agent, DMPP as a photo-initiator, and KTpClPB from Sigma-Aldrich. The salts material used are Pb(NO3)2, KCl, NaCl, NH4Cl, Tris-HCl, MgCl2.6H2O, CaCl2.2H2O, CuCl2.2H2O, Cd(NO3)2.4H2O, HCl 36% solution and also the solid of NaOH obtained from Merck.
Experimental
Synthesis and characterization of p(THFA-co-HEMA) CP
Initially, p(THFA-co-HEMA) CP were prepared by mixing THFA monomers, HEMA, DMPP and HDDA monomers based on the composition as provided in Table 1. Then the mixture is homogeneously mixed until clear solution is formed.
Table 1: Variations in the composition of p(THFA-co-HEMA) CP.
CP | THFA (mg) | HEMA (mg) | HDDA (mg) | DMPP (mg) |
---|---|---|---|---|
TH9.50.5 | 95 | 5 | 0.016 | 1 |
TH91 | 90 | 10 | 0.016 | 1 |
TH82 | 80 | 20 | 0.016 | 1 |
TH73 | 70 | 30 | 0.016 | 1 |
TH64 | 60 | 40 | 0.016 | 1 |
Each composition was placed on the microscope glass slide and PP process was carried out for 3.5 min, under continuous N2 atmosphere in the UV-exposure box. The thin film formed on the surface of the glass slide was then slowly removed and stored in a sample bottle for structural analysis using FTIR. In addition to structural testing, Tg testing was also carried out using a TGA/DSC device in the formed film. Furthermore, films that met Tg value from -40 to 15 were selected as sensing polymer for Pb-ISE sensor fabrication.
Pb(II)-ISE preparation and performance test
The following are the steps of preparing Pb-ISE sensor. Firstly, Ag/AgCl electrode is used as the sensor platform. The Ag/AgCl electrode is produced through electroplating technique in 0.5M KCl solution for 30 sec. Ag-SPE platform is connected to the positive (+) terminal and the Pt RE is connected to the 9 V battery negative (-) terminal. After the electroplating process is completed, the Ag-SPE surface forms a brownish-brown AgCl thin layer. Next, the formed Ag/AgCl electrode is washed with deionized water and dried with tissue paper.
The second preparation step is to form an inner pHEMA layer on the surface of the prepared Ag/AgCl electrode. The formation of this inner layer was carried out by dripping 0.1 L of the HEMA monomer mixture on the surface of the Ag/AgCl electrode and then the PP process is carried out for 1.5 min, in a UV-exposure box with continuous N2 gas flow. After the PP process, a thin pHEMA film layer is formed which then further hydrated by a droplet of 0.01M Pb(NO3)2 solution, for 15 min. After the hydration process is completed, then the remaining solution is wiped gently with tissue paper.
In the third sensor preparation step, 100 L of each variation of the selected CP composition is mixed with 1 mg of KTpClPB liphophilic salt and Pb Ionophore (IV) with a 1:1 mole ratio between KTpClPB: Lead Ionophore (IV). Then the mixture is vigorously mix until a clear liquid mixture is formed. 3 L of each CP mixture is drop coated on the surface of the pHEMA layer and subsequently a PP process is carried out for 3.5 min, in N gas flow atmosphere. After the PP process has completed, a new p(THFA-co-HEMA)CP film layer is formed as a sensing layer and the Pb-ISE sensor is ready for characterization. The Pb-ISE sensor is conditioned in a 0.01 M Pb(NO3)2 solution for 30 min. The Nernstian numbers and the linear range of measurements are carried out. The best characterized CP composition will proceed for performance tests such as selectivity coefficient, pH effect test, and validation test. In this study, validation testing is carried out by measuring the Ct of artificial solutions and real samples using the Pb-ISE sensor and comparing it with the AAS standard method with SNI number 06-6989.8-2004.
Results and discussion
Structure analysis of p(THFA-co-HEMA) CP
The newly synthesized p(THFA-co-HEMA) CP sensing structural analysis was further characterized before further being used as sensing host matrix. This novel acrylate CP based has never been reported before. In this study, structural analysis was carried out using FTIR. The sample used in this study was the TH82 CP sample as a representative of the five variations in the composition of the synthesized acrylate polymer. This is because, in principle, the molecular structure of the five variants of the acrylate CP used has the same structure. The analysis results of the p(THFA-co-HEMA) CP structure can be seen from Fig. 2.
Based on Fig. 2, it appears that the FTIR spectrum produces seven peaks in the region of 1400-4000 cm-1 which is the main area. In addition, there are also seven peaks in the area below 1400 cm-1 which is a fingerprint area that serves as supporting data for FTIR analysis 27. In the area of 3426 cm-1, there is a broad peak and this is a stretching band of -OH functional groups. In addition, the -OH group is also seen at the peak of 1636 cm-1 which is the bending band of -OH groups. Meanwhile, in the area of 2800-3000 cm-1, there are sharp twin peaks at positions 2943 and 2864 cm-1. Both peaks are stretched from asymmetric and symmetrical C-H groups derived from methylene and methyl. At position 1721 cm-1 there is a very sharp peak, where this peak is a stretching of the C=O functional group. In an area of around 1400 cm-1 there are two peaks, where the first is in position 1416 cm-1 which is C-H bending from the methyl group and secondly at position 1410 cm-1 which is the-methyl group. The functional group of the -methyl group is also seen on the band 811 cm-1. In the areas, 1269 and 1171 cm-1 are the bending of the -C-O-C. Meanwhile, at the 994 cm-1, the C-O originates from the furan ring. The 1062, 982, and 884 cm-1 bands are typical of the acrylate band 28-33.
Based on the characterization that was obtained from FTIR analysis, as previously described above, CP structure formed from the PP process with UV light looks like Fig. 3.
DSC analysis
A membrane with Tg mechanical properties of novel p(THFA-co-HEMA) was determined using DSC analysis. A significant Tg value was important to minimize fouling tendency of the developed Pb-ISE sensor. This was due to the generated Tg value that affects the movement of ions in the membrane used during the Pb ion measurement process 2,6-8. In this study, DSC measurements were carried out on five composition variances and carried out with a temperature range of -30oC to 100oC, where the results of the DSC test are tabulated in Table 2. Meanwhile, the heating rate used in this DSC test is 10oC/min. Sorting the temperature range of the DSC test is based on the Tg values of each THFA and HEMA are -13 and 87 oC 34-35. So, this causes the Tg value of the synthesized acrylate CP to be in the Tg range of both monomers. This condition is in accordance with Fox equation, which explains that Tg value of CP will be proportional to the weight fraction (ω) of each monomer 34,36-37.
Table 2: Tg values of variations IN p(THFA-co-HEMA) CP which is prepared by using PP technique.
Membrane | THFA (mg) | HEMA (mg) | Tg estimation (o C) | Tg measurement (o C) |
---|---|---|---|---|
TH9.50.5 | 95 | 5 | -8 | -10.5 |
TH91 | 90 | 10 | -3 | -6.5 |
TH82 | 80 | 20 | 7 | 5.1 |
TH73 | 70 | 30 | 17 | 17.7 |
TH64 | 60 | 40 | 27.2 | 26.3 |
Based on Table 2, it can be seen that Tg generated from DSC measurements did not differ greatly from the results of calculations based on eq. (1). It also shows that the presence of HEMA monomers caused changes in Tg value of CP. The measurement results show the higher HEMA amount, the higher Tg value of resulting CP and in accordance with the predictions of Fox equation. This is inseparable from the influence of -CH3 derived from HEMA monomers 28. Based on test results, membranes TH9.50.5, TH91 and TH82 were selected for fabrication as a Pb-ISE sensor, since they have Tg value required for ISE sensors, which is from -40 to 15 ºC 2,6-8.
Response of Pb-ISE
In a potentiometric technique, an ISE sensor membrane WE is coupled with a standard RE to form a complete sensor measurement system. In principle, potentiometric analysis measures V that occurs between an ISE sensor with a RE vs. the logarithm of ion activity under conditions without the presence of a current. Measurement of potentiometric techniques will follow the phenomenon of the Nernstian equation, as in eq. eq (2):
where E is the difference between ISE sensor and RE, R is the universal gas constant with a value of 8.314 J / K mol, T is the absolute temperature at 298 K. Meanwhile F is a Faraday number of 96,435 C/mol, the zA is the A ion charge and aA is A ion activity 38. In this study, the A ion used was Pb2+ ion.
In evaluating the Pb-ISE sensor response, a total of three replicate sensors have been fabricated from each formulated CP variants namely TH9.50.5, TH91 and TH82. All Pb-ISE electrodes are tested in Pb(NO3)2 solution in the range from 0.1 to 10-9 M. Based on the result shown in Table 3, all CP for Pb-ISE sensors displayed Nernstian number of 28.2-29.7 mV/dec, which is close to the theoretical Nernstian number of divalent ions of 24.6 - 34.6 mV/Dec 2,39. Therefore, Pb-ISE sensors response can be depicted from eq. (3). Fig. 4 shows that sensor responses did not significantly change at lower Ct from 10-6 to 10-9 M Pb2+.
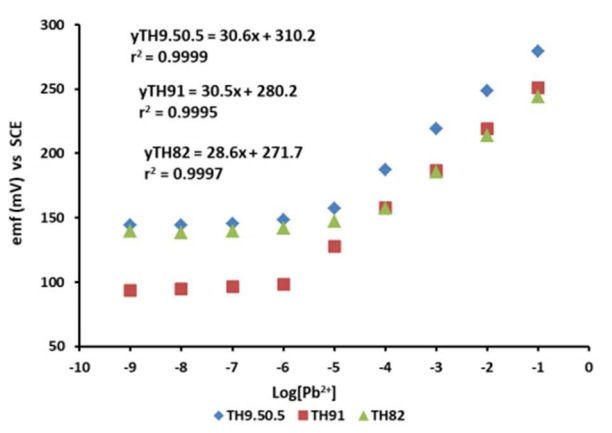
Figure 4: Pb-ISE response of three different P(THFA-co-HEMA) CP ratios in the Ct of Pb(NO3)2 solution.
Meanwhile, in the area below the LOD for each sensor, the response of the ISE has been seen to be constant even though there is a change in the Ct of the test solution used. This LOD region is calculated based on the point of intersection between the linear range region and the constant region 40. This is due to the fact that the number of ions at low Ct is increasingly limited 2, but also due to the hydrophilicity of the membrane. This hydrophilicity will affect the movement of ions in the membrane 25,40-41.
Table 3: Performance of Pb-ISE sensor with variations in the type of membrane P(THFA-co-HEMA) CP with variations in Ct of Pb(NO3)2 solution.
Membrane | Slope (mV/dec) | Linear range (M) | LOD (M) | R2 |
---|---|---|---|---|
TH9.50.5 | 30.6 | 0.1 - 10-5 | 3.80 x 10-6 | 0.9999 |
TH91 | 30.5 | 0.1 - 10-6 | 7.59 x 10-7 | 0.9995 |
TH82 | 28.6 | 0.1 - 10-4 | 2.40 x 10-5 | 0.9997 |
Although all three sensors have good Nernstian numbers, they sensors exhibited different linear range as shown in Table 3. Synthesized TH91 sensing membrane had the longest linear range among the three membrane types, followed by TH9.50.5, while the shortest was TH82. This linear range difference was influenced by the presence of HEMA monomers in the structure of acrylate polymers. In principle, HEMA is a hydrophilic acrylate-based monomer with a Tg that is higher than THFA monomer of 87 ºC 34-35. With these characteristics, the presence of HEMA monomers at a certain ratio will cause variance response in Pb-ISE sensors 25.
On the other hand, the sensor with TH9.50.5 membrane has shown a linear response in the range from 0.1 to 10-5 M. Similar findings have been reported by previous researchers, who only have used pTHFA membrane for the development of Pb-ISE sensor 2. The is mainly due to the content of 5% w/w HEMA monomer which is too low. Hence, it is not enough to increase the wide linear range of Pb-ISE sensor. Meanwhile, the presence of a 10% w/w HEMA monomer in TH91 membrane caused the Pb-ISE sensor range to increase from 0.1 to 10-6 M. This increase was due to the hydrophilic nature of the HEMA monomer, which also changed CP. This change is due to ions diffusion from the sample, which penetrate more easily into the membrane located on the sensor surface to be responded by the Ag/AgCl transducer used in this study 25.
Meanwhile, the presence of HEMA monomers as much as 20% w/w actually caused the linear range of Pb-ISE sensor to decrease from 0.1 to 10-4 M. This is not independent of the acrylate CP being increasingly hydrophilic. This condition has not only caused more Pb2+ ions to penetrate the sensor, but also a small number of interfering ions and counter ions, which, in turn, will affect the Pb-ISE sensor response 25,42. In addition, this increasingly hydrophilic nature causes components in the membrane such as ionophore and lipophilic salts to be released more easily to the sample solution. This situation disrupts the balance of components in the membrane, which is one of the important factors in the fabrication of ISE sensors 2. Based on data shown in Fig. 4 and Table 3, Pb-ISE sensor with HT91 membrane was selected for further testing.
Perfomance of Pb-ISE
In this study, the optimum sensor result obtained from TH91 membrane was further tested. This is based on the fact that the TH91 membrane had more extensive measurement range than that of other two types of membranes. The detail performance testing of Pb-ISE sensor included testing the coefficient of selectivity, response time and pH effect. Selectivity test describes the ability of sensor to respond to the target analyte without being influenced by the presence of contaminant ions in the test sample. This test is crucial, considering that real samples generally consist of various types of ions 44,46. There are several methods for testing selectivity coefficient, such as MSM and SSM 13,46-48. SSM selectivity test method is performed due to its simplicity and rapid procedure. The test is done by measuring V value of Pb2+ ion, and also measuring V of each of the interfering ions such as K+, Na+, NH4 +, Mg2+, Ca2+, Cu2+ and Cd2+, at the Ct of 0.1 M 49. Furthermore, the selectivity coefficient value is calculated using coefficient selectivity eq. 4, and the results are shown in Table 4.
where EB is E value of the confounding ion, EA is the primary ion E, zA is the primary ion charge, zB is the interfering ion charge, F is the Faraday number of 96,485 C/ mole, R is the universal gas constant of 8.314 J/K mole, T is the temperature at 25oC (298 K) and finally aA is the target ion Ct 2.
Table 4: Coefficient selectivity (LogKpot a,b) of Pb-ISE sensor towards interfering ions (n = 3).
Interfering ion | LogKpot a,b |
---|---|
K+ | -6.53 ± 0.11 |
Na+ | -6.35 ± 0.16 |
NH4 + | -6.76 ± 0.11 |
Mg2+ | -12.15 ± 0.13 |
Ca2+ | -12.12 ± 0.14 |
Cu2+ | -4.33 ± 0.11 |
Cd2+ | -6.45 ± 0.17 |
Based on the selectivity test from Table 4, Pb-ISE sensor incorporated with p(THFA-co-HEMA) shows good selectivity against other interfering cations, which makes it advantageous as membrane matrix for potentiometric sensor and results in high sensitivity toward Pb2+. The sensing matrix is incorporated with lead ionophore (IV) which is used for Pb2+ ion recognition in this study. Lead selective ionophore (IV) is derived from amides which makes this type of ionophore to have superior selectivity even in the presence of interfering cations. This result is in agreement with reports from literatures in lead sensor development 2,16,37,50-52.
Meanwhile, response time is the time acquired by the Pb-ISE sensor to respond to changes in sample Ct instantly until it reaches an equilibrium point following the Le Chartlier principle. This equilibrium point is an indication that the Pb-ISE sensor has reached its stability point marked by changes <1 mV/min 2,40,52. Hence, this test is conducted by measuring V from Pb-ISE sensor in a solution of Pb(NO3)2 at initially low Ct of 0.001 M and sequentially, the sample solution is injected with a solution of Pb(NO3)2 with a Ct of 0.1 M to achieve final Ct of 0.01 M. In Fig. 5, at T = 0 or at the beginning of the measurement, the sensor has a E value of 217.7 mV and after T = 30 sec, the reading is 217.6 mV. The result shows that during the first 30 s, the Pb-ISE sensor showed a fairly good stability even after 30 s of being left idling to run in 0.001 M test solution. Later, the test solution was injected with 0.1 M Pb(NO3)2, at 32.3 sec, and the sensor E value instantaneously changed from 218.5 mV, until a new equilibrium point was achieved at 39 s, with a final E value of 246.0 mV. So, the calculated response time required for the Pb-ISE sensor is 6.7 sec with the increased E value of 27.5 mV. The obtained response time is quite good and proportional to the response time provided by the Pb-ISE sensor that has been developed by previous researchers 14-15,17,53. Good response time was inseparable from the low Tg and the hydrophilicity of the synthesized p(THFA-co-HEMA) membrane. It has conclusively been shown that Pb2+ ions are easily diffused from the sample onto the surface of sensor Ag/AgCl transducer which is shown in Fig. 1 2-6,25,42.
The main challenge faced by researchers in the development of Pb-ISE is that the metal formation is strongly influenced by pH 2. In this study, pH testing is carried out at pH 2-10 using variations of three Ct of Pb (NO3)2 solution with Ct between 0.1-10-3 M as shown in Fig. 6. It can be seen from Fig. 6, that overall sensor response in different Ct of Pb(NO3)2 shows that pH 2 has a slightly decrease spike of E value compared to that at pH 3-8. Meanwhile, at pH 3-8, the E value of the resulting Pb-ISE sensor is relatively no different. However, at pH 9-10, there is a change in the E value signal in all the test solutions used. The condition observed at pH<3 is due to the Lead Ionophore (IV) used in the sensor formulation which underwent a deprotonation process that affects Pb-ISE sensor response 12,50,54. Meanwhile, when the pH>8, the environmental conditions of the sample became more alkaline. This results in some of Pb ions turned into Pb(OH)2 deposits. The formation of these deposits causes the Ct of Pb2+ ions in the sample to decrease. This reduction in the Ct of Pb2+ ions certainly had an impact on decreasing the E value 14,18,53. These findings suggest that the developed Pb-ISE sensor from the p(THFA-co-HEMA) membrane effectively works in the pH range from 3 to 8.
In general, Pb-ISE based on p(THFA-co-HEMA) membrane shows comparable performance to some of the Pb-ISE that have been developed by several previous researchers as can be seen in Table 5.
Table 5: Comparison of Pb-ISE performance based on p(THFA-co-HEMA) membrane with previous studies.
Slope (mV/dec) | Dynamic range (M) | LOD (M) | pH range | response time (s) | ref |
---|---|---|---|---|---|
25.79 | 0.1 - 10-6 | 4 x 10-7 | 5 -7.2 | 10 | 14 |
26.49 ± 0.7 | 0.1 - 10-6 | 7.6 x 10-7 | 4 -7 | <10 | 15 |
29.0 ± 0.2 | 2x10-3 - 2x10-9 | 4 x 10-10 | 2 - 4.8 | <10 | 16 |
27.65 | 0.1 -10-7 | - | 3 - 7 | 10 | 17 |
30 ± 0.2 | 0.1 - 10-5 | 4 x 10-6 | 4 - 8 | 45 | 55 |
29.8 | 0.1 - 10-8 | 4.3 × 10−9 | 3.5 - 7.3 | 5-7 | 56 |
29.1 ± 0.3 | 10−3 to 10−9 | 6.31× 10−10 | 3 - 9 | <5 | 57 |
31.5 | I0-2 - 10-8 | 6.0 × 10−9 | 2 - 8 | 5 | 58 |
27.25 | 10-5 -10-10 | < 10-10 | 7 - 8 | 25 | 59 |
30.5 | 0.1 - 10-6 | 7.59 x 10-7 | 3 - 8 | 6.7 | this work |
Validation test
Electrochemical sensor validation test is one of the most well-known methods to ensure the developed Pb-ISE sensor performance is comparable to standard methods 14. The standard method used in this study is AAS, as recommended by SNI 06-6989.8-2004 2. This validation test uses two types of samples. The first sample is artificial, with Ct of 10, 50 and 100 ppm, and the second one was collected from CREW and sediments.
Sediment samples from CREW were selected because CR is one of the main rivers that divides the city of Jakarta which flows from the Mount Gede Pangrango to the Jakarta Bay. Along with the flow of the CR, there are many urbanization community activities that dispose trash into the river, including battery waste that contains a lot of heavy metals such as Pb. In addition, along the river flow, especially those approaching the estuary area many industrial activities also dispose their waste into the river 60-62. The result of the sensor validation test in artificial samples and real samples are summarized in Table 6. In this study, real samples used were spiked with a standard solution of 50 ppm.
The result indicates that detection of Pb2+ ion using Pb-ISE sensor is comparable to those obtained using AAS standard method accordance to SNI 06-6989.8-2004 procedure. For real water sample, it shows that in the presence of other ions Pb-ISE sensor does not significantly affect the measurement result. However, the real sample data that is produced provides an indicator that the condition of CREW quality has exceeded the standard water quality of 10 g/L 2,9. This condition is due to the extreme process of urbanization and industrial activities that occur along CR flow causing waste discharge accumulations in the area. This data is also comparable with the results from previous studies which stated that the content of heavy metals such as Pb in CREW was from 8.49 to 50.96 ppm, and in CRMS was from 20 to 336 g/g 60. The findings of this research provided insight about the river water quality and have raised serious concern on Jakarta’s local government.
Table 6: Comparison of the measurement results of Pb2+ ions on artificial samples and real samples using Pb-ISE sensors and AAS (n = 3).
Samples | Pb-ISE | AAS |
---|---|---|
10 ppm | 10.9 ± 0.2 | 10.7 ± 0.4 |
50 ppm | 52.1 ± 1.3 | 52.5 ± 0.9 |
100 ppm | 103.3 ± 0.5 | 102.6 ± 0.7 |
CREW (ppm) | 9.3 ± 1.9 | 9.2 ± 2.0 |
CRMS g/g) | 275.4 ± 10.3 | 277.3 ± 4.0 |
Conclusions
The acrylate of p(THFA-co-HEMA) CP was successfully synthesized and characterized using FTIR. This CP was applied as a supporting membrane matrix in the fabrication of Pb-ISE sensors. From the five variations of proposed p(THFA-co-HEMA) CP compositions, only three variations met the specifications for Pb-ISE sensors development, which included TH9.50.5, TH91 and TH82, with Tg values from -10.5 to 5.1 ºC. The best composition of three CP was TH91, within a range from 0.1 to 10-6 M and LOD reaching of 4.16 x 10-7 M. The diffused Pb-ISE sensor showed good selectivity coefficients for several types of ions such as K+, Na+, NH4+, Mg2+, Ca2+, Cu2+ and Cd2+, in the pH range from 3 to 8. Besides that, Pb-ISE sensor had fairly good response time of 6.7 s for 27.5 mV. The sensor test results with artificial solutions and real samples from CRMS were comparable to AAS’s standard method, according to SNI 06-6989.8-2004.
Acknowledgments
The authors thank to Universitas Mercu Buana for funding this research through the KLN research scheme with grant number 02-5/646/B-SPK/III/2020. Acknowledgments were also given to the collaborating Institutions who participated in the completion of this research such as the Universiti Kebangsaan Malaysia who helped with the FTIR analysis, Mimos Berhad who assisted with FTIR testing, and LIPI Fisika who assisted with Tg analysis using DSC tools.
Authors’ contributions
Sagir Alva: in research acted as the head of the research project and is in charge of designing research, writing, data analysis and conducting correspondence with publishers. Rika Sri Utami: played a role in the synthesis and characterization of the poly(THFA-co-HEMA) membrane. Melny Andrian: played a role in optimizing membrane composition and conducting performance testing of Pb-ISE in this study. Arsyad Widinugroho: played a role in preparing real samples and conducting validation tests. Aiman Sajidah Abd Aziz: played a role in assisting the DSC analysis. Siti Aishah Hasbullah: helped to analyze the performance of the Pb-ISE sensor; assisted in writing the manuscript. Deni Shidqi Khaerudini: helped in analyzing FTIR. Dafit Feriyanto: helped to write the draft of the article and in administrating this research. Kee Shyuan Loh: helped designing the synthesis and characterization of membranes in this study. Edy Herianto Majlan: assisted the validation testing process.
Abbreviations
AAS: atomic absorption spectrophotometry
CaCl2.2H2O: calcium chloride, dihydrate
Cd(NO3)2.4H2O: cadmium nitrate tetrahydrate
CP: co-polymer
CR: Ciliwung river
CREW: Ciliwung river estuary water
CRMS: Ciliwung river mouth sediments
Ct: concentration
CuCl2.2H2O: copper(II) chloride dehydrate
DMPP: 2,2-dimethoxy-2-phenylacetophenone
DSC: differential scanning calorimetry
E: potential
FTIR: Fourier-transform infrared spectroscopy
HDDA: 1,6-hexanediol diacrylate
HEMA: 2-hydroxyethyl methacrylate
ISE: ion selective electrode
KTpClPB: potassium tetrakis(4-chlorophenyl)borate lipophilic salt
LOD: limit of detection
MB: methyl methacrylate-butyl acrylate
MgCl2.6H2O: magnesium chloride hexahydrate
MSM: mixed solution method
NH4Cl: ammonium chloride
NSA: National Standardization Agency
pBA: polybutyl acrylate
pBA-HEMA: butyl acrylate-hydroxy ethyl methacrylate
Pb(NO3)2: lead(II) nitrate
PHXA: poly(hydroxamic acid)
pIDA: poly isododecyl acrylate
PP: photo-polymer/photo-polymerization
PVC: polyvinyl chloride
R2: correlation coefficient
RE: reference electrode
SC: saturated calomel
SNI: Indonesian National Standard
SPE: screen-printed electrode
SSM: separated solution method
T: temperature
Tg: glass transition temperature
TGA: thermal gravimetric analysis
THFA: tetrahydrofurfuryl acrylate
THFA-co-HEMA: tetrahydro furfuryl acrylate-hydroxyl ethyl methacrylate
TP: thermo-polymerization
V: potential difference
WE: working electrode
WFD: Water Framework Directive
WHO: World Health Organization