Introduction
PA
PA is a pathogen that can cause severe and even deadly acute or chronic infections. This gram-negative bacterium is classified under the Pseudomonas genus, and is also known as Pyocyanin bacillus, blue pus Bacillus or Pyocianica1.
PA infections
PA infections can affect any part of the body such as: respiratory system (pneumonia); heart (endocarditis); central nervous system (meningitis); ears (otitis); eyes, in cornea (keratitis and conjunctivitis, which can even cause total blindness); bones and joints; digestive tract (gastroenteritis); urinary tract infections, which are most often due to hospital instrumentation; and skin (dermatitis and folliculitis).
PA resistance to antibiotics
PA is naturally resistant to antibiotics, since it has poor permeability to antibacterial agents, and it also generates enzymes that can degrade antibiotic molecules, like cephalosporins.
Treatment of pseudomonal infections
Antibiotics that are effective against PA include carbapenems, certain fluoroquinolones (ciprofloxacin, at high doses), some penicillin types (piperacillin-tazobactam) and third-generation cephalosporins (ceftazidime) (Table 1).
Table 1: PA sensitivity to antibiotics 3.
Antibiotic | Sensitivity | Intermediary | Resistance |
---|---|---|---|
Ticarcillin | 54.5% | 14.7% | 30,8% |
Ceftazidime | 78.6% | 11,6% | 9,8% |
Imipenem | 72.2% | 12% | 15,8% |
Ciprofloxacin | 60,4% | 2,3% | 37,3% |
Amikacin | 75,3% | 10,1% | 14,6% |
Since PA is resistant to antibiotics, infections should be treated by combined therapy 2. Pseudomonal infections are typically treated using a combination of two antimicrobial agents: beta-lactam and aminoglycoside. Nevertheless, appropriate treatments may depend on the type of infection and affected organs 3, and surgical intervention is rarely needed.
Since antibiotics generally used to treat PA infections have side effects on health, there is the the need to seek an alternative.
MO
MO is a versatile, highly adaptable, fast-growing tropical tree that is native to Asia, but also cultivated in Africa, and Central and South America. It belongs to the Moringa family, which includes thirteen species (order: Brassicales). In recent times, MO has become one of the most important products in pharmaceutical industry 4. Historically, MO various parts have been traditionally used as food and/or in traditional medicine. Its seeds are known to alleviate the effects of various diseases, particularly inflammatory-mediated conditions, such as cardiovascular and gastrointestinal illnesses, since they have anti-inflammatory, antioxidant, hypotensive, antibacterial and chemo preventive properties 4-6. Phytochemicals derived from MO seeds have been linked to various bioactivities, including GLS, ITC, nitriles, carbamates and thiocarbamates presence 5-7. Bioactive peptides derived from natural plant proteins have recently gained attention in various industries. Lately, natural plant proteins have been extensively sought in health, food preservation and pharmaceutical industries, due to bioactive peptides presence. These peptides typically consist of a chain with 2 to 20 amino acid residues 7,8. MO has a reputation as a bactericidal plant, and does not show any chronological toxicity 11.
Material and methods
Reagents and chemicals
All chemicals used in this work are of high quality. Gr powder, (spectroscopic grade RWB, Ringsdorff-Werke GmbH, Bonn-Bad Godesberg, Germany), was obtained from Aldrich, and used without further purification. The mother electrode modifying reagent, used in the form of MO powder, was obtained from a Cameron medicine tree. PA bacteria strain is of commercial grade. Deionized water was used to prepare all the solutions.
Device
Ec experiments were conducted using a Voltalab potentiostat (model PGSTAT 100, Eco Chemie BV, Utrecht, The Netherlands), driven by general purpose Ec systems data processing software (Voltalab master 4 software). The measurement cell used in this work was a three-electrode cell. CPE-MO was used as WE, SCE served as RE and Pt plate as AE.
Results and discussion
Fig. 1 shows CV recorded at a SR of 50 mV/S, on the CPE surface (curve a) and on the CPE-MO (curve b) surface, respectively, in 1 M NaCl.
The two voltammograms show different shapes, which proves that the mother electrode modification was successful. CPE-MO shows a small oxidation peak at around 0.5 V, and a large peak towards the cathode, at about -0.5 V. The two peaks are sufficiently aligned, indicating that this is not a redox system.
The morphology of the elaborated electrodes surfaces was characterized by SEM. The SEM image of CPE (Fig. 2 (A)) shows a compact surface which presents some defects and relatively deep pores. CPE surface has an irregular structure, with reliefs. CPE-MO surface shows reliefs and porous connections. The pores are very deeply surrounded by large MO surfaces (Fig. 2 (B)).
Fig. 3 (A) represents CV in the absence (curve a) and presence of C₆H₁₂O₆ (curve b). We can notice that C₆H₁₂O₆ oxidation was manifested by an anodic peak, which appeared around 0.9 V, towards the anode. This well-defined peak led to MO reduction peaks disappearance, which suggests that its addition to CPE promoted C₆H₁₂O₆ oxidation. C₆H₁₂O₆ oxidation on the CPE-MO surface remarkable changed its morphology, which seems to consist of large branched rods with deep pores between them (Fig. 3. B).
PA addition to 1 M NaCl with C₆H₁₂O₆ affected the CV (Fig. 4 (A)). The CV shows that redox peaks disappeared, and C₆H₁₂O₆ oxidation was deactivated. These phenomena occurred because the bacteria adhered on to the electrode surface and blocked its active sites. SEM image confirms these observations, and shows a homogeneous and regular structure, without the previously seen batons (Fig. 4 (B)).
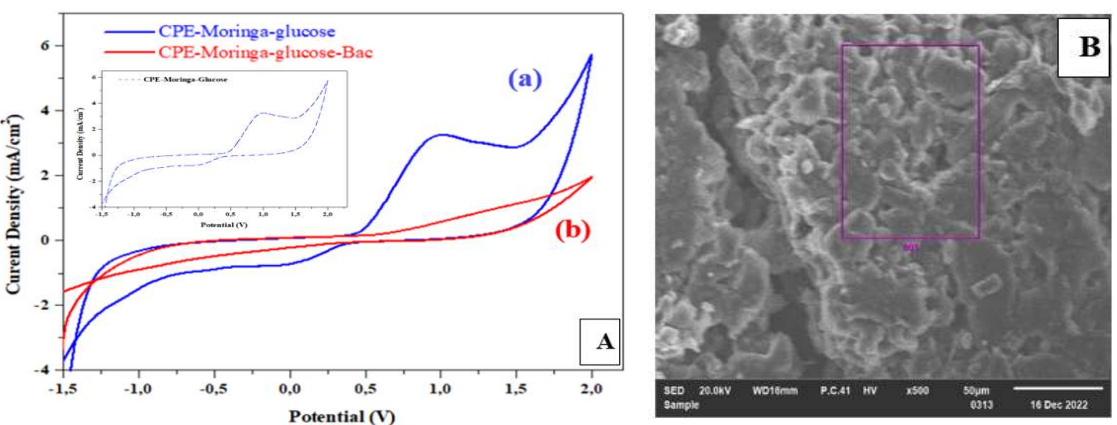
Figure 4: Effect of PA in 1 M NaCl with C₆H₁₂O₆ on (A) GCE-MO, at SR of 50 mV/s-1 (CV); and (B) CPE-MO (SEM image).
Ct of PA did not affect the CV (Fig. 5) recorded on the modified CPE-MO surface, in 1 M NaCl with C₆H₁₂O₆. On the contrary, j values decreased with higher Ct of PA, which confirms the hypothesis that it formed a film on the CPE-MO surface, and blocked its active sites.
EIS diagrams in the Nyquist plane (Fig. 6) show the bacteria Ct effect on C₆H₁₂O₆ oxidation, at the CPE-MO surface. It is seen that EIS curves have the shape of semi-circles, for all Ct of PA. These curves moved towards low frequencies, which confirms CPE-MO surface conductivity loss. Ec parameters deduced from EIS curves are shown in Table 2. Capacitance values increased considerably with the Ct of PA, which confirms that they formed a dense film on the CPE-MO surface.
Table 2: Ec parameters from EIS.
PA (µl) | Diameters (ohm/cm2) | Correlation | R1 (ohm/cm2) | R2 (ohm/cm2) | R2-R1 | Ct (µf/cm2) |
---|---|---|---|---|---|---|
0 | 22.2 | 0.998 | 587.2 | 19.65 | 567.55 | 80.99 |
50 | 44.1 | 0.986 | 115.9 | 35.69 | 80.21 | 294 |
100 | 44 | 0.983 | 119.2 | 39.23 | 79.97 | 288.7 |
150 | 55.7 | 0.986 | 121.2 | 46.54 | 74.66 | 243.4 |
200 | 58.6 | 0.979 | 127.2 | 50.85 | 76.35 | 197.7 |
250 | 62.2 | 0.980 | 124.4 | 52.16 | 72.24 | 217.2 |
To explain C₆H₁₂O₆ oxidation peak disappearance, the polarization curve of CPE-MO in NaCl with PA (Fig. 7) was plotted.
The curve processing allowed to determine Ec parameters (Table 3) such as j and E (I = 0). PA addition to NaCl shifted the equilibrium E towards very negative values, giving relatively lower CR, which supports the hypothesis that a PA film was formed on the CPE-MO surface, hindering C₆H₁₂O₆ oxidation.
Table 3: Ec parameters from polarization curve.
PA (µl) | E (I = 0) (K/ohm/cm2) | Rp (K/ohm/cm2) | j | (a (mV) | (c (mV) | R2 | CR (mm/y) |
---|---|---|---|---|---|---|---|
50 | -519.1 | 1.30 | 28.63 | 263.5 | -199.4 | 0.998 | 334.8 |
100 | -535.6 | 1.37 | 26.51 | 262.2 | -195.6 | 0.998 | 314.8 |
150 | -554.9 | 1, 39 | 26.17 | 261.2 | -190.9 | 0.997 | 306 |
200 | -555.7 | 1.42 | 27.30 | 279.1 | -200 | 0.997 | 319.3 |
250 | -561.9 | 1.45 | 25.48 | 266.8 | -193 | 0.998 | 298 |
300 | -545.1 | 1.46 | 26.17 | 263.8 | -194.9 | 0.998 | 294.4 |
350 | -570.2 | 1.49 | 24.87 | 268.3 | -194.1 | 0.998 | 290.9 |
CA study of C₆H₁₂O₆ oxidation kinetics
The transients presented in Fig. 8 show a remarkable activity of CPE-MO, concerning CH₁₂O₆ oxidation, at the reaction start, for the first 20 min. j decreased as a function of time. These transients were recorded at glycose oxidation E. J values recorded on the plateaus gradually decreased, which means that CPE-MO activity was not affected by PA (Fig. 9).
Conclusion
A CPE-MO was herein developed. Its performance was tested by CV, upon C₆H₁₂O₆ oxidation. The CV recorded at the CPE-MO surface showed a well-defined peak, which was due to C₆H₁₂O₆ Ec oxidation. PA addition to NaCl with C₆H₁₂O₆ eliminated its oxidation peak. The hypothesis that PA would adhere to the CPE-MO surface, blocking its active sites and, subsequently, C₆H₁₂O₆ oxidation, was proposed. To verify this hypothesis, polarization curves and EIS, which revealed the presence of a non-conductive film on the CPE-MO surface, were employed.
Authors’ contributions
Oubaouz Mohamed: processed data manipulation. Niraka Blaise: processed data manipulation. Abdelilah Chtaini: analyzed data and addressed the article premise.
Abbreviations
AE: auxiliary electrode
C₆H₁₂O₆: glucose
CA: chronoamperometry
CPE: carbon paste electrode
CR: corrosion rate
Ct: concentration
CV: cyclic voltammetry
E: potential
Ec: electrochemical
EIS: impedance spectroscopy
GCE: graphite carbon electrode
GLS: glucosinolates
Gr: graphite
I: current
ITC: isothiocyanates
j: current density
NaCl: sodium chloride
PA: Pseudomonas aeruginosa
R2: coefficient of determination
RE: reference electrode
Redox: reduction/oxidation reaction
Rp: polarization resistance
SCE: saturated calomel electrode
SEM: scanning electron microscopy
SR: scanning rate
WE: working electrode
Symbols definitions
βa: anodic Tafel slope
βc: cathodic Tafel slope