INTRODUCTION
High-intensity interval training (HIIT) is characterised as a time-efficient intervention to improve aerobic conditioning and body composition in a few training sessions (Chin et al., 2020). A large number of studies have shown that HIIT contributes to increase cardiorespiratory capacity, muscle power and endurance and can be used to optimise performance in various sports (Vasconcelos, Protzen, Galliano, Kirk, & Del Vecchio, 2020), as well as in the prevention and treatment of non-communicable chronic diseases (Martin-Smith et al., 2020).
There is an increase in the production of reactive oxygen species (ROS) during physical training due to moments of ischemia and reperfusion of the musculoskeletal system, although even in a resting state, these muscle cells continue to produce ROS continuously (Powers & Schrager, 2022).
ROS are responsible for causing oxidative damage to biomolecules, changing their functional properties; however, it is known that the production of free radicals (FR) is fundamental for the cell signalling processes responsible for inducing physiological adaptations (Bouviere et al., 2021). Although the human organism has a complex endogenous antioxidant defence system, which neutralises FR, cells can present physiological disorders, such as apoptosis, due to the irreparable damage they cause to DNA (Luo et al., 2019).
Excess FR, such as ROS and reactive nitrogen species (RNS), alter redox homeostasis by an imbalance of the antioxidant and oxidant system, leading to damage to macromolecules, such as lipids, proteins and DNA, while there is disruption of the signalling redox, resulting in a condition called oxidative stress (Sies & Jones, 2020). In this sense, this study aimed to analyse the effect of 15 HIIT sessions using a swimming protocol on the redox state of Wistar rats.
METHODS
Animals
Wistar rats weighing 250 g – 350 g, obtained from the Sectorial Animal Facility of the Department of Physiology (DFS), Universidade Federal de Sergipe-UFS, were used. Rats were randomly housed in appropriate cages under controlled temperature (22± 3°C) and 12 h light/dark cycle. Animals had free access to specific rodent food and filtered water. The present work was approved by the Ethics Committee on the Use of Animals at UFS (protocol 15/2017). Animals were divided into 2 groups (n= 10): Control Group (CG): composed of animals that did not perform the HIIT protocol and Training Group (TG): composed of animals that underwent the HIIT protocol.
Procedures
HIIT Protocol
The training intensity was defined by the load, and the literature demonstrates that loads above 6% of the animal's body weight in swimming protocols are considered high-intensity exercise (Cunha et al., 2009; Gobatto et al., 2001).
Animals were submitted to a swimming exercise, for which black PVC cylinders (120 cm deep and 80 cm in diameter) were used, with water at a depth of 50 cm and an average temperature of 25± 2°C, with weights fixed in a vest located on the animal's back. To perform the exercise, animals were individually placed in PVC cylinders with water. Animals were acclimatised in the aquatic environment with swimming sessions lasting 20 min without load, 3 times, the week before the beginning of the training protocol. The HIIT protocol was in accordance with Terada et al. (2001), which consisted of 10 to 14 swimming periods lasting 20 seconds and intervals of 10 seconds between each period, performed during 15 consecutive sessions. After 24 hours of the end of the experimental protocol, animals were adequately anesthetised to collect blood, muscle (gastrocnemius), liver and heart tissue samples for analysis.
Biochemical analyses
Animals were anaesthetised with ketamine/xylazine (75 mg/kg + 10 mg/kg i.p) (Flecknell, 2009) and blood (± 5 mL) was collected by cardiac puncture and then euthanised by exsanguination under anaesthesia. After collection, blood was immediately centrifuged at 4,000 x g for 15 min at ± 4°C, and the supernatant was stored at ± −70°C. In parallel, organs (muscle, liver and heart) were removed and washed 3 times with 1.15% potassium chloride (KCl) solution, dried and weighed. Subsequently, they were homogenised where each gram of tissue was mixed with 5 mL of KCl + 10 μL of phenylmethylsulfonyl fluoride (PMSF — 100 mmol. L-1) + 15 μL of 10% Triton solution and centrifuged at 3,000 x g for 10 min at ± −70°C for further analysis of oxidative stress markers.
In vivo determination of TBARS
Lipid oxidation was determined by measuring thiobarbituric acid reactive substances (TBARS) according to the method described by Lapenna, Ciofani, Pierdomenico, Giamberardino and Cuccurullo (2001). For this, 200 μL aliquots (blood and organs) were added to a 400 μL mixture formed by equal parts of 15% trichloroacetic acid (TCA), 0.25 N HCl and 0.375% TBA, plus 2.5 mM of butylated hydroxytoluene (BHT) and 40 μL of 8.1% sodium dodecyl sulfate (SDS), being heated for 30 min at 95°C in an oven. The mixture pH was adjusted to 0.9 with concentrated HCl. BHT was used to prevent lipid peroxidation during heating. After cooling to room temperature and adding 4 mL of butanol, the material was centrifuged at 800 xg for 15 min at ± 4 °C, and the supernatant absorbance was measured at 532 nm. The molar extinction coefficient used was 1.54 x 10 5 M −1 cm −1, and the TBARS result was expressed in nmol Eq MDA/mL of serum for blood samples or nmol Eq MDA/mL of serum for organs.
Determination of total sulfhydryls (thiols)
The determination of groups was performed according to the methodology described by Faure and Lafond (1995), in which 50 μL aliquots (blood and organs) were mixed in 1 mL of tris-EDTA buffer, pH 8.2. Then, the first reading (A) was performed in a spectrophotometer at 412 nm. After reading, samples were transferred to test tubes and mixed with 20 μL of 10 mM DTNB diluted in methanol (4 mg/mL), left to stand in the dark. At the end of 15 min, the second absorbance reading (A2) was performed. The SH concentration was calculated according to Equation 1:
The result was expressed in nmol.mg-1 tissue sulfhydryl.
Statistical analysis
Data obtained were expressed as mean and standard deviation (SD). To observe data normality, the Shapiro-Wilk test was used. To assess the significance of the differences between means, the Student's t-test was used, followed by Bonferroni's post-test. In order to verify the effect size, Cohen's d test was used, in addition to the cut-off points 0.2 to 0.5 for a small effect, 0.5 to 0.8 for a medium effect, and greater than 0.8 for a large effect, according to Lakens (2013). Values were considered statistically significant when p< 0.05. GraphPad Prism statistical software version 7.0 (GraphPad Software, San Diego, CA, USA) was used for all these procedures.
RESULTS
The HIIT protocol used in the present study proved to be efficient in inducing oxidative damage in animals by measuring the malondialdehyde concentration (MDA) (Figure 1), and an increase of 305.6% was observed in the MDA production in the serum of TG animals (14.49± 1.78 nmol EqMDA/Ml; p< 0.001), in relation to CG animals (3.57± 1.33 nmol EqMDA/mL) and the effect was large (Cohen's d= 6.95), as shown in Figure 1A.
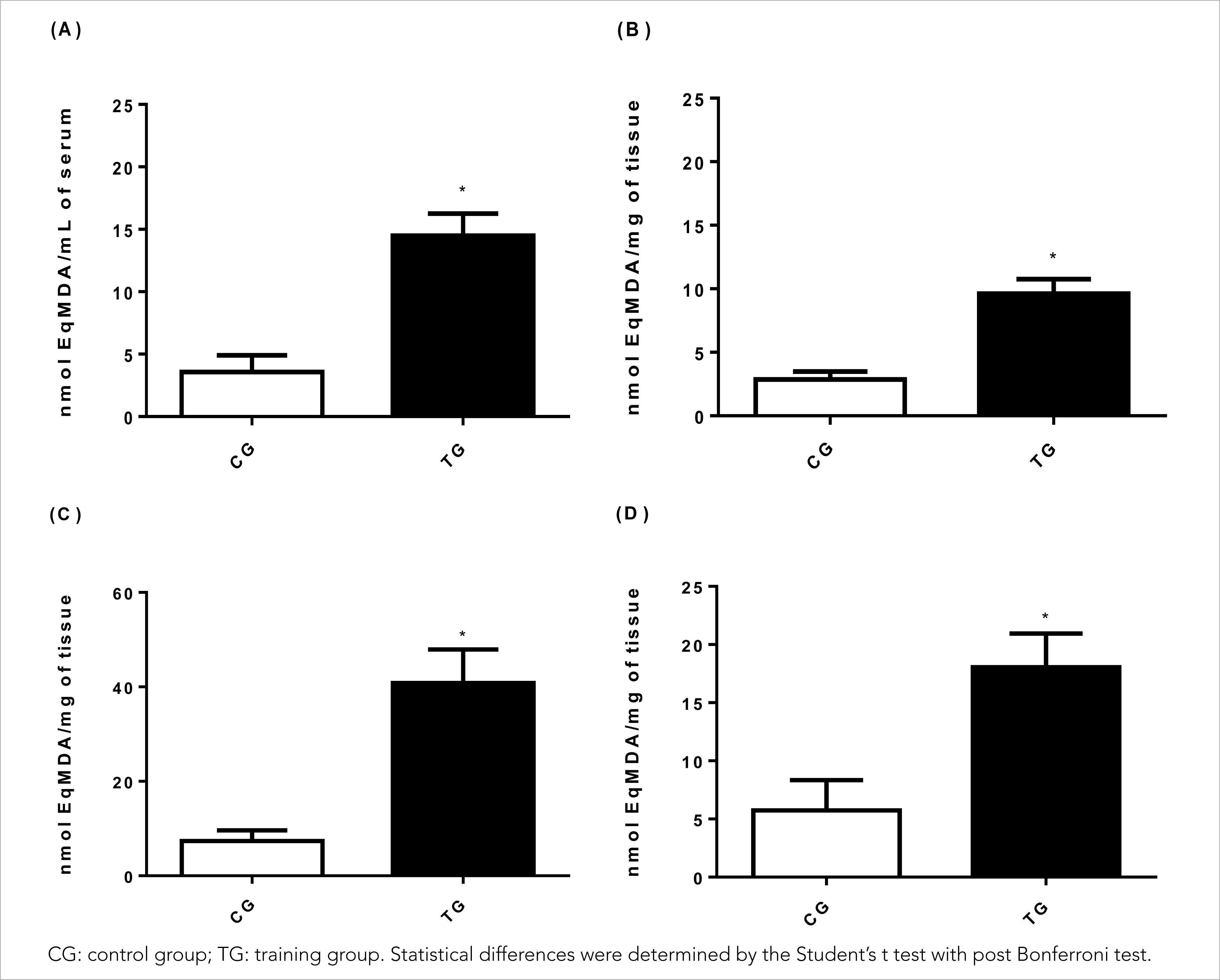
Figure 1 Effects of HIIT on (A) serum malondialdehyde (MDA) concentration, (B) muscle tissue (gastrocnemius), (C) liver tissue and (D) cardiac tissue.
Regarding the muscle tissue (Figure 1B), a significant increase of 259.2% in the MDA concentration was observed in TG animals (9.61± 1.61 nmol EqMDA/mg) in relation to CG animals (2.85± 0.63 nmol EqMDA/mg) (p< 0.001) with a large effect (Cohen's d= 5,53). In parallel, in liver tissues (Figure 1C), HIIT significantly increased lipid peroxidation by 452.6% in TG animals (40.85± 7.05 nmol EqMDA/mg) in relation to CG animals (7.40± 2.39 nmol EqMDA/mg) (p< 0.001) and the effect was large (Cohen's d= 6.39).
Regarding oxidative damage to lipids in cardiac tissues (Figure 1D), the present study demonstrated that HIIT in TG animals (18.06± 2.88 nmol EqMDA/mg) was significantly effective in inducing lipid peroxidation by 215% compared to CG animals (5.73± 2.60 nmol EqMDA/mg) (p< 0.001) with a large effect (Cohen's d= 4.49).
The content of sulfhydryl groups (Figure 2) evaluated in the present study indirectly indicates the recruitment of the glutathione antioxidant defence system of the tested tissue. However, the system was widely used in TG animals (148.84± 24.66 nmol/mL), with a significant reduction by 40% in relation to sérum (242.80± 35.85 nmol/mL) (p< 0.001), and the effect was large (Cohen's d= 3.05) (Figure 2A).
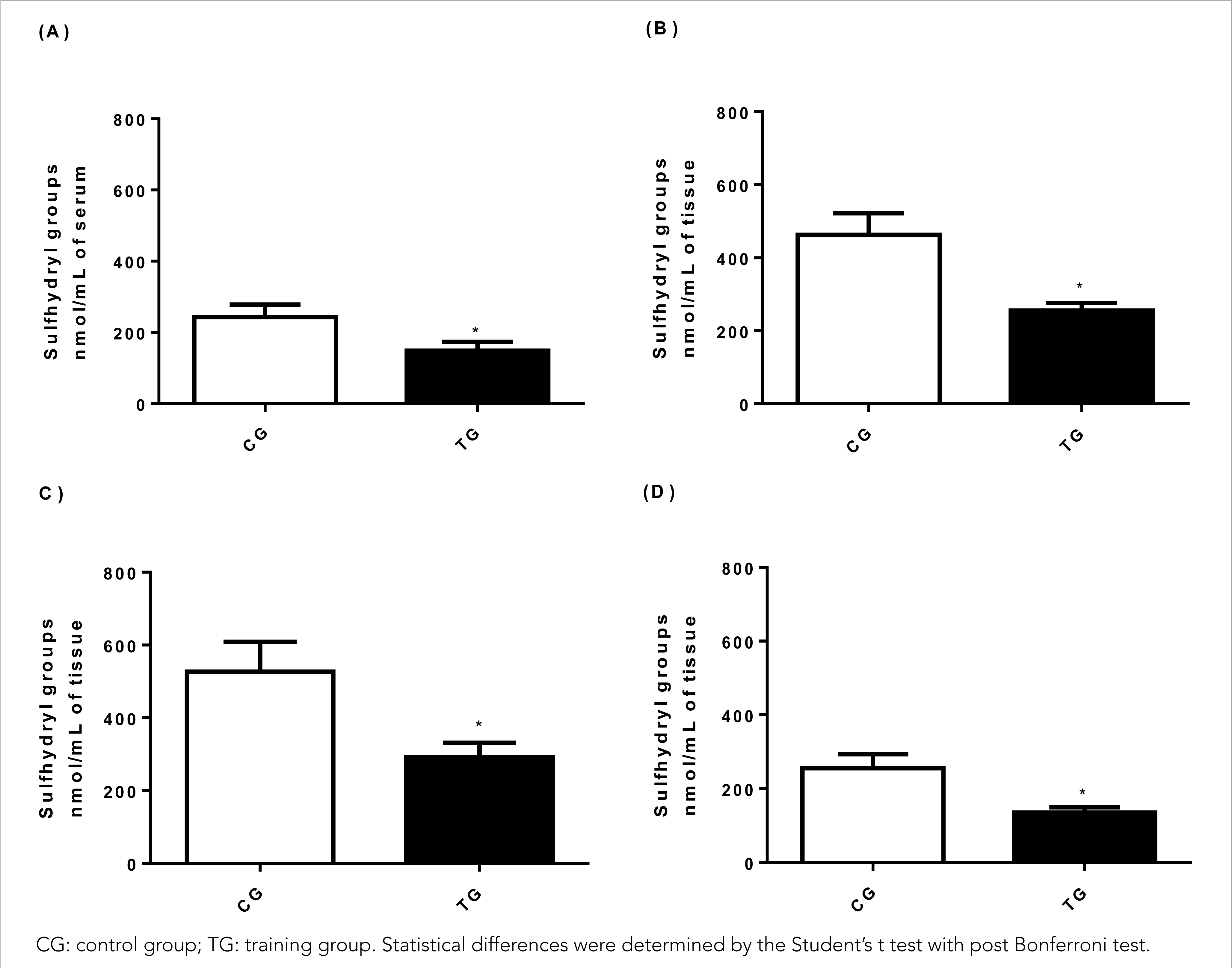
Figure 2 Effect of HIIT on the concentration of sulfhydryl groups in (A) serum, (B) muscle tissue (gastrocnemius), (C) liver tissue, (D) cardiac tissue.
Concerning muscle tissue (Figure 2B), TG animals (255.75± 20.47 nmol/mg) showed a 44.7% decrease in total sulfhydryl groups when compared to CG animals (462.86± 59.64 nmol /mg) (p< 0.001) and the effect was large (Cohen's d= 4,65). While in the liver tissue (Figure 2C), a reduction of 44.6% in TG animals was observed (291.92± 39.66 nmol/mg) compared to CG animals (537.07± 82.17 nmol/mg) (p< 0.001) with a large effect (Cohen's d= 3.80) demonstrating the action of HIIT on thiol groups.
In the cardiac tissue (Figure 2D), a significant reduction of 47.2% in sulfhydryl groups was observed in TG animals (134.83± 15.14 nmol/mg) when compared to CG animals (255.71± 37.79 nmol/mg) (p< 0.001) and the effect was large (Cohen's d= 4.20).
DISCUSSION
The present study demonstrated that HIIT increased oxidative damage markers in Wistar rats in 15 consecutive sessions. Variations in intensity, type and duration of exercise directly influence ROS production, and consequently, oxidative damage and HIIT seem to result in greater oxidation of lipids in the liver, muscle and blood (Dos Santos et al., 2021; Vieira-Souza et al., 2021).
According to Pillon Barcelos, Freire Royes, Gonzalez-Gallego, and Bresciani (2017), chronic exercise models increase liver-related stress. Delwing-de Lima et al. (2018), Dos Santos et al. (2014), and Souza et al. (2020) used a high-intensity exercise protocol in animals and identified that it induced oxidative stress, corroborating the findings of this study.
One of the stimuli that generate ROS is the activation of the NADPH oxidase complex, which can be stimulated by the activation of 28 cytokines in the Nf-Kb and Ik-B signalling pathways. Gomes et al. (2016) skeletal musculature consumes large amounts of oxygen, increasing the sources that generate ROS, characterising the presence of oxidative stress, a factor that may also have contributed to the gastrocnemius muscle to being one of the tissues with the highest lipid oxidation in the present study.
Unlike the findings by De Araujo, Papoti, Dos Reis, de Mello and Gobatto (2012) and Songstad et al. (2015), who submitted rats to high-intensity training with water jumping and treadmill running, where the levels of antioxidant enzymes remained significantly unchanged compared to the control, showing insignificant changes in ROS, in the present study, a significant reduction in sulfhydryl groups was observed in the group submitted to training when compared to the control group.
Malondialdehyde (MDA) is an excellent biomarker for assessing lipid peroxidation under different physiological and pathological situations (Mas-Bargues et al., 2021). The assessment of lipid damage is positively related to pathological conditions such as neurological diseases, cardiovascular disease, diabetes and cancer (Ghonimi, Elsharkawi, Khyal, & Abdelghani, 2021; Kangari et al., 2018; Klisic et al., 2018). In the present study, a significant increase in this marker was observed, in agreement with Freitas et al. (2019), who demonstrated an increase in MDA of rats submitted to a HIIT protocol on the treadmill for six weeks compared to untrained rats. This demonstrates that the presence of MDA can vary not only according to the physiological condition but also to the tissue under evaluation. Some studies have demonstrated that the elevation of MDA and other oxidative damage markers after a training period are associated with an increase in the antioxidant defence system (Heiat, Heiat, & Shojaeifard, 2021).
Animals submitted to a swimming HIIT protocol seemed to have H2O2 production increased in the mitochondria after 6 weeks of training (Ramos-Filho et al., 2015) since excess of H2O2 inside the cell involves increased production of the OH• radical and, consequently, increased irreversible oxidative damage to lipids, DNA and proteins (Kruk, Aboul-Enein, Kładna, & Bowser, 2019; Tretter, Hochreiter, Zach, Krenn, & Klein, 2022).
Although a shorter training period was used in the present study, the frequency between sessions was also lower, thus reducing the recovery time between them, which allowed for greater stress in animals. In contrast, Stanojevic et al. (2016) observed that the decrease in recovery time between continuous swimming sessions did not result in oxidative stress in rats’ heart and blood tissue after 12 weeks of training. However, the animals performed the entire training period without additional load and underwent an adaptation period of 8 weeks until the overtraining induction protocol was carried out, which consisted of 3 weeks. In contrast, in the present study, animals underwent an acclimatisation period followed by training with a load. However, oxidative stress has been shown to be associated with overtraining since the accumulation of ROS within the skeletal muscle can promote the loss of muscle contraction force due to reduced intramuscular calcium release (Cheng, Jude, & Lanner, 2020). However, HIIT was shown to have a beneficial effect on the redox state of obese rats, but this effect was specific to some tissues (Groussard et al., 2019).
Proteins that contain thiol groups (-SH) conserved in cysteine residues are sensitive to redox reactions; the sulfhydryl groups in the side chain of these proteins are susceptible to electron transfer reactions (Chen & Tang, 2021). The sulfhydryl groups refer to the use of the glutathione antioxidant defence system. The glutathione molecule has in its structure the thiol group responsible for numerous functions in the biological system, having greater importance in the antioxidant defence system (Finaud, Lac, & Filaire, 2006). Animals submitted to a high-fat diet associated with high-intensity interval training showed reduced sulfhydryl groups in plasma; however, these changes were not observed in the liver (Delwing-De Lima et al., 2018). In the present study, all tissues under analysis showed a significant reduction of this marker.
In fact, the role of ROS produced during exercise is still controversial regarding their real impacts on health and performance, as well as the absence of specific biomarker indices for monitoring the redox state in the population (Theofilidis, Bogdanis, Koutedakis, & Karatzaferi, 2018). However, we know that the oxidative response varies according to the type of exercise, intensity, duration, training time and nutritional status (Powers et al., 2020). A possible limitation would be the fact that only two biomarkers were used. Furthermore, future research should increase the number of consecutive sessions of the training protocol.
CONCLUSIONS
Therefore, in the present study, it was observed that 15 sessions of a HIIT swimming protocol promoted oxidative damage in serum and muscle, cardiac and hepatic tissues of Wistar rats, however, further studies are needed to explore new training protocols in order to observe the behaviour of other biomarkers, as well as the possible intracellular and molecular mechanisms involved in the control of the redox state.