Introduction
The materials most commonly used to manufacture dental implants and the abutments that connect the implant to the prosthetic crown are titanium and different titanium alloys.
These are biocompatible materials with good mechanical properties and a low risk of corrosion.1 On the other hand, zirconia abutments, first introduced in 1997, have aesthetic properties superior to titanium and high mechanical strength.2,3 However, zirconia is much harder than titanium, and zirconia abutments directly screwed to the implant are suspected of wearing the implant platform, leading to loss of geometry and causing instability at the implant-abutment connection.4
Implant-abutment connections can be classified as external or internal depending on the presence or absence of a geometric index extending above the implant’s coronal surface.5 The external-hexagon connection consists of a 0.7-mm high hexagon extending above the implant’s coronal surface.6
This connection, introduced by Brånemark, has the advantage of providing long-term follow-up data and compatibility among multiple implant systems.5 However, its hexagon’s low height implies a reduced contact area between the abutment and the implant platform, causing high stresses to the screw that connects the abutment to the implant. Thus, this connection is associated with an increased incidence of screw loosening.5,6
On the other hand, in internal connections, the indexing mechanisms extend into the implant, increasing the abutment-implant contact area and reducing the stresses applied to the prosthetic screws.5 Internal connections have a lower incidence of screw loosening, better joint strength,5,7 and improve the implant’s ability to withstand axial loads compared to external-hexagon connections.8 Several types of internal connections are currently available on the market, with varying geometries, and the main types are internal hexagon, tri-channel, crossfit, hexagonal conical, and cone morse.5
The implant-abutment connection’s stability has been identified as a major factor in the long-term success of dental implants.4 Micromovements between the abutment and the implant create an unstable connection that may lead to biological and prosthetic complications.4 Low amplitude oscillatory movements can cause implant fretting wear, which may widen the microgap between the abutment and implant, increasing bacterial infiltration and bone loss.9,10 The increased implant abutment instability also creates rotational freedom that may lead to a higher incidence of screw loosening.8
However, few studies evaluate micromovements between the implant and the abutment with different connections, and the abutment material’s effect has not been adequately reported in the dental literature. Therefore, the present research study aimed to evaluate the effects of the abutment material and the connection type on the implant-abutment connection’s displacement amplitude and rigidity when subjected to cyclic loads. The following null hypotheses were tested: 1) the abutment material (titanium or zirconia) does not affect the implant-abutment connection’s rigidity; 2) the connection type (external-hexagon, tri-channel, or conical connection) does not affect the implant-abutment connection’s rigidity.
Material and methods
The sample size n=3 was calculated based on a pilot study. A power analysis indicated statistical significance (α=0.05) at 80% power.11,12The study evaluated eighteen implants with three different connection geometries: external hexagon (Brånemark MK III TiUnite RP Nobel Biocare, Göteborg, Sweden), tri-channel (Replace Tapered RP Nobel Biocare, Göteborg, Sweden), and conical connection (Nobel Active RP Nobel Biocare, Göteborg, Sweden). The implants were inserted in the center of epoxy resin blocks (DPC-Laminierharz LT 2, Duroplast- Chemie Vertriebs GmbH, Neustadt/Wied, Germany) according to the manufacturer’s recommended surgical protocols.
Half of the implants were connected to zirconia abutments (Zirkon Translucence, Zirkonzahn GmbH, Gais, Italy) and the other half to grade-5 titanium abutments (Titan5 Zirkonzahn GmbH, Gais, Italy), forming six study groups.
The abutments were designed in the software Zirkonzhan (Zirkonzhan Modelier, Gais, Italy) and produced with CADCAM techniques (Zirkonzahn M5, Gais, Italy). They had a 5-mm wide, 8-mm height, and 30º inclination of the incisal edge.13
This 30º slope was designed to create an accurate contact with the hemispherical brace of the testing machine.13 The abutments were directly screwed to the respective implants using grade-5 titanium screws recommended by the manufacturer, specific for titanium or zirconia abutments (Abutment screw, Zirkonzhan, Gais, Italy). A torque of 35 Ncm was applied with a manual torque wrench (Nobel Biocare, Göteborg, Sweden).
The specimens were submitted to 1,200,000 load cycles,2,13 with a sinusoidal load varying between 100 N and 10 N (load amplitude of 90 N),2 at a frequency of 10 Hz in a fatigue testing machine (Instron ElectroPlus E10000). During all the tests, the specimens were submerged in artificial saliva (SAGF).14 According to the ISO 14801:2007 standard,13 cyclic loads were applied with an angulation of 30º to the implant’s long axis (Figure 1).
The fatigue tests were carried out with load control. During load application, the software that controls the mechanical fatigue testing machine graphically recorded, for each load cycle, the abutment-implant connection’s maximum and minimum displacement. The displacement amplitude was recorded as the difference between each cycle’s maximum and minimum displacement. The amplitude of the applied load was constant at 90 N.
For each load cycle, the rigidity was obtained using the following formula:15,16 Rigidity =Force amplitude (N) Displacement amplitude (mm) In the analysis of rigidity evolution throughout the cycles, three different periods stand out during a given test (Figure 2). The first period corresponds to the first 100 cycles and is a phase of stabilization and accommodation of the abutment in contact with the load sphere; this period was discarded (Figure 2-A). The second period, between the 100th and 2000th cycles, corresponds to a stage of reduced rigidity variation (Figure 2-B). The third period, from the 2000th cycle to the end of each test, corresponds to a period of great rigidity variation. The second and third periods’ average rigidity were calculated and designated as initial rigidity and final rigidity, respectively. The average total rigidity for each specimen was calculated based on the second and third periods’ rigidity (Figures 2-B and 2-C).
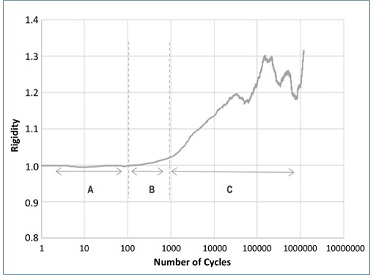
Figure 2 Exemplificative graph for the externalhexagon titanium specimen, showing rigidity evolution with the number of cycles during the mechanical test. Three different periods stand out: (A) Initial period of adaptation of the load control of the machine; (B) Period of reduced rigidity variation (Initial Rigidity); (C) Period of marked rigidity variation (Final Rigidity).
The dependent variable was the total rigidity (continuous variable, values expressed in N/mm), and the independente variables were the abutment material and connection type. Data were analyzed statistically using SPSS Statistics, version 28.0.1 (IBM SPSS Statistics, New York, United States).
After checking normality (Shapiro-Wilk tests; p>0.05) and the homogeneity of variances (Levene test; p>0.05), the 2-way ANOVA followed by Tukey’s post-hoc tests were used to detect the effect of main factors. A confidence level of α=0.05 was assumed.
Results
The average initial and total rigidity values of the various groups and the variation in rigidity (maximum and minimum) are shown in Table 1. In all groups, the average total rigidity was higher than the initial, representing an increase in rigidity throughout the test. The total rigidity values varied between a maximum of 2098.7 N/mm for the tri-channel connection with a zirconia abutment and a minimum of 1367.2
N/mm for the external-hexagon connection with a zirconia abutment.
There were no statistically significant differences (p=0.883) in the abutment material’s effect on the rigidity of the implant- abutment connection (Table 2). Figure 3 represents the different abutment materials’ mean total rigidity and respective standard deviation values.
Table 2 Measures of central tendency and dispersion of total rigidity, according to abutment material (N/mm), n=9. The average values have the same letter due to not showing statistically significant differences (p>0.05).

Conversely, the different groups showed statistically significant differences (p=0.013) in the connection type’s effect on the implant-abutment connection rigidity (Table 3). The two-way ANOVA test demonstrated no interaction between the two variables - type of connection and abutment material (p=0.156). The post-hoc tests revealed statistically significant differences between the total rigidity of the different connection types. Namely, there were differences between the external-hexagon and the tri-channel connections (p=0.016) and between the external-hexagon and the conical connections (p=0.036). There were no differences between the tri-channel and the conical connections (p=0.897). Figure 4 shows the values of the different connection types.
Table 3 Measures of central tendency and dispersion of total rigidity, according to connection type (N/mm), n=6. Connections with the same letter do not show statistically significant differences (p>0.05).

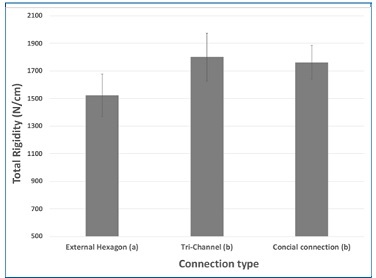
Figure 4 Influence of the connection type on the total rigidity (n=6). Connections with the same letter do not show statistically significant differences (p>0.05).
Discussion
In the present study, a mechanical testing machine measured the abutment displacement during cyclic loads to calculate the abutment-implant connection rigidity. The main conclusions of this work were that the abutment material did not influence the rigidity of the implant-abutment connection (the first null hypothesis cannot be rejected), but the connection type did (the second null hypothesis rejected).
The external-hexagon connection’s rigidity was lower than the tri-channel and conical internal connections, with statistically significant differences. These results can be related to the implant-abutment contact area, which differs according to the connection type. A connection with a larger contact area has more resistance to micromovements and higher joint stability and rigidity. Thus, a connection’s stress
concentration reduces as the internal area of the platform increases.17,18 The contact area between implant and abutment is higher in internal connections than in the external- hexagon connection.19 In external connections, the axial loads occur directly over the screw.7 On the other hand, internal connections have higher stability, transfer forces more deeply, and tend to dissipate less stress on the screw than the external hexagon.7 The incidence of screw loosening is 5.4% with internal connections,20 while it varies between 6 to 48% with the external-hexagon connection.7
However, two clinical studies that evaluated clinical complications between implants with the external-hexagon and internal connections during 5 years in function did not detect statistically significant differences between the two types of connection.21,22 Another clinical study evaluated two internal connections for 1 year after the placement of prosthetic rehabilitation and also found no differences between the two types of internal connections.23 Regarding the abutment material, contrary to the results obtained in the present study, zirconia abutments were reported to have superior rotational freedom than titanium abutments with external connection implants (Brånemark, Nobel Biocare).24
The authors found no studies assessing the rigidity of different abutment-implant connections in the literature, but some studies measured the micromovements of the abutment-implant connection using various methodologies.
As in the present study, other authors argue that the type of connection conditions the micromovements between the abutments and the implants.25,26 The tri-channel abutments seem to be the connection with the smallest magnitude of micromovements, which can be attributed to its geometry.
The tri-channel connection abutment25,26 and Morse taper15 have lower displacements when compared to the external-hexagon connection. However, contrary to the presente study, some authors report that the conical connection (Nobel Active, Nobel Biocare) presented a higher displacement than the external-hexagon.25 This study results indicate a general tendency for an increase in rigidity with the progression of the test since the average total rigidity of all groups was higher after the application of cyclic loads, i.e., compared to the initial rigidity obtained in an initial phase of the tests.
During the cyclic loading, a zirconia abutment with a conical connection fractured. This specimen has since been replaced. The fracture occurred at the base of the abutment and may result from a smaller thickness of the zirconia in this area.27
Power analysis was made to calculate the sample size, and the ISO standard defines that data must be generated for a load cycle diagram, in which at least three specimens survive and none fail during the test.13 However, a larger sample would be convenient because small sample sizes and few specimens per group may influence the interactions between main factors.
Another limitation of the study is that only a few studies with heterogenous methodologies discussed this subject. Further studies with the same methodologies (number of cycles, frequency, artificial saliva) are needed. Moreover, ISO digital protocols should be created to standardize the comparing tools. A clinical trial with real oral conditions is also importante to deepen the knowledge of this phenomenon.