Serviços Personalizados
Journal
Artigo
Indicadores
Links relacionados
Compartilhar
Revista de Gestão Costeira Integrada
versão On-line ISSN 1646-8872
RGCI vol.13 no.4 Lisboa dez. 2013
https://doi.org/10.5894/rgci430
ARTICLE / ARTIGO
Cholinesterase activity as biomarker of neurotoxicity: utility in the assessment of aquatic environment contamination*
Actividade da colinesterase como biomarcador de neurotoxicidade: avaliação da contaminação em ambientes aquáticos**
Jamel Jebali@, I; Sana Ben KhedherI; Marwa SabbaghI; Naouel KamelI; Mohamed BanniI; Hamadi BoussettaI
@Corresponding author: jebali.jamel@laposte.net; jamel.jebali@fsb.rnu.tn
ILaboratory of Biochemical and Environmental Toxicology, Higher Institute of Agriculture, Chott-Mariem, 4042-Sousse, Tunisia
ABSTRACT
Cholinesterase can take place in aquatic organisms under a series of environmental adverse conditions. The study of cholinesterases in these organisms can give important information about their physiological status and about environmental health. However, it is very important to know how the environmental factors such as fluctuation of physicochemical parameters associated to the presence of pollutants might affect these cholinesterase activities. We studied the response of cholinesterase activity in the caged cockle Cerastoderma glaucum. In addition, we evaluated the potential uses of cholinesterase activity in the common sole, which inhabit the Tunisian coast, subjected to different stress conditions, such as the exposure to different contaminants. This review summarizes the data obtained in some studies carried out in organisms from the Tunisian aquatic environment.
Keywords: Cholinesterases, Environmental stress, Biomonitoring, Aquatic Environments.
RESUMO
A colinesterase está presente nos organismos aquáticos em condições de stress ambiental. O estudo da colinesterase fornece informações acerca da condição fisiológica e saúde ambiental. Contudo é importante averiguar de que modo os factores ambientais, tais como a flutuação dos parâmetros físico-químicos, associados à presença de poluentes afectam a actividade das colinesterases. Neste trabalho são apresentadas os níveis de actividade da colinesterase em Cerastoderma glaucum. São ainda apresentados os potenciais usos da actividade das colinesterases na solha comum que habita a costa Tunisina. Este trabalho sumariza também os resultados obtidos até agora em outros organismos estudados na Costa Tunisina.
Palavras-Chave: Colinesterases, Stress Ambiental, Biomonitorização, Ambientes Aquáticos.
1. INTRODUCTION
The initial interaction between chemicals and biological systems occurs at the cellular level. Thus, cells may be expected to react to chemical exposure. The assessment of chemicals toxicity at cellular level makes cellular responses a suitable tool for the early and sensitive detection of chemicals exposure (Monserrat et al., 2007).
Chemical pollutants may pass the membrane by either special transport processes including active transport, facilitated transport, endocytosis, or passive diffusion (Helmut and Braunbeck, 1998). Having crossed the cell membrane, chemicals may exert their toxicity by different ways resulting in altering cellular structural and function as it is shown in figure 1. As a determinant of intracellular chemical toxicity, biotransformation of chemicals plays a key role, since many chemicals are not toxic, mutagenic or carcinogenic per se, but require metabolic activation to reactive species that can interact with target macromolecules in the cell (Livingstone, 1998). An example of chemicals that exert their toxicity by link to cellular molecules is the organophosphorous and carbamates pesticides that may be irreversibly linked to cholinesterase enzymes and in particular to acetylcholinesterase leading to neurotoxicity effects.
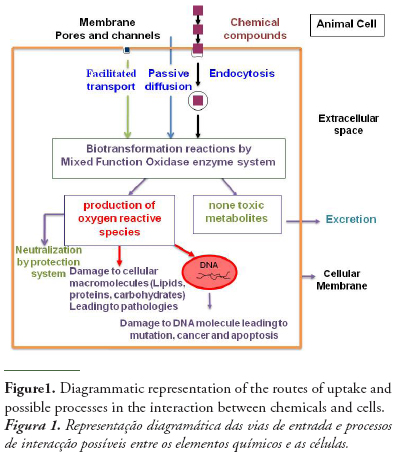
2. CHOLINESTERASES: STRUCTURE AND FUNCTIONS
Cholinesterases (ChEs) are enzymes widely distributed in nature from microorganisms and protozoans to vertebrates, including human. They have been extensively studied since they are highly polymorphic enzymes in most species, play an important role in the transmission of nervous influx and are the specific target for most nerve agents and insecticides. The number of genes coding for ChEs varies according to species (Forget & Bocquené, 1999). There are two basic types of ChEs: acetylcholinesterases and butyrylcholinesterase (BChE) are the best known and characterized (Massoulié et al., 1993). Both types of ChEs are evolutionary related, but are encoded in different genes and have their own specific features (e.g. substrate specificity, kinetic parameters, etc.) allowing for them to be discerned from one another. AChE is a specialized enzyme and its main physiological function is hydrolysis of acetylcholine (ACh), a mediator of neurotransduction in cholinergic synapses. BChE is less specific in relation to substrate specificity for hydrolysis and its physiological role remains unclear. Kinetic properties of ChEs can be determined from their amino acid composition and the conformation of their active center. Acetycholinesterases are a homo- and betero-oligomeric molecular forms, which belongs to the α/β proteins class. Each monomer is established by 12 β-strands surrounded with 15 α –helices (Sussman et al., 1991). AChEs are two types of oligomeric forms. The first is a dimeric and tetrameric association of subunits covalently linked by disulfide bonds. These molecular forms are called globular AChEs G1, G2 and G4. The second type of AChE consists of one, two or three tetrameric of subunits associated with a collagen tail and establishes the asymmetric forms of AChE named A4, A8, and A12 (Massoulié et al., 1993).
2.1. Cholinesterases of vertebrates: acetylcholinesterase and pseudocholinesterases
Vertebrates possess two cholinesterases, acetylcholinesterase and butyrylcholinesterase. The first is synthesized in hematopoiesis, occurs in the brain, endplate of skeletal muscle and erythrocyte membrane, and its main function is to regulate neuronal communication by hydrolyzing the ubiquitous neurotransmitter acetylcholine in synaptic cleft (Quinn, 1987; Silman & Sussman, 2005). The second is synthesized in liver and is present in plasma, smooth muscle, pancreas, adipocytes, skin, brain and heart (Çokugras, 2003). Although its physiological function is not well defined, BChE is pointed as one of the main detoxifying enzymes able to hydrolyze or scavenge a broad range of xenobiotic compounds like cocaine, heroin, anaesthetics, and pesticides (Soreq & Zakut, 1990; Taylor, 1991; Çokugras, 2003; Nicolet et al., 2003). Some studies hypothesized that one of the functions of BChE is to protect AChE against anticholinesterasic agents (Whitaker, 1980; Whitaker, 1986). Pezzementi & Chatonnet (2010) reported that ChEs emerged from a family of proteins with adhesion properties. Both play other roles in the neuronal tissue, particularly in neuronal differentiation and development, cell growth, adhesion and signaling. In addition, AChE participates even in hematopoietic differentiation (Chatonnet & Lockridge, 1989; Taylor, 1991; Johnson and Moore, 2000; Silman & Sussman, 2005). Moreover, AChE and BChE are different concerning several other aspects: while AChE has an in vivo half-life of 120 days, BChE lasts 7-12 days. AChE is inhibited by substrate excess and BChE is activated by substrate excess (Lopez-Carillo & Lopez-Cervantes, 1993; Çokugras, 2003). AChE is selectively inhibited by propidium, DDM, caffeine, Nu1250, 62c47 and BW284c51 while BChE is selectively inhibited by percaine, isopestox, ethopropazine, Iso-OMPA, bambuterol and haloxon (Chatonnet & Lockridge, 1989; Harel et al., 1992; Kovarik et al., 2003). BChE has a larger space in its active site, which can hydrolize or be inhibited by a range of compounds. AChE has a more specific active site (Çokugras, 2003).
Some of these features are governed by crucial differences in the structure of the enzymes such as: 1) the difference in size of active site can be explained by six aromatic residues lining the active site of AChE that are missing in BChE; 2) two of these (Phe-288 and Phe-290) are replaced by leucine and valine, respectively, in BChE. This feature prevents the entrance of butyrylcholine in the AChE active site; 3) peripheral site specific-ligands such as propidium does not inhibit BChE because the residue Trp-279, which is part of the peripheral anionic site located at the entrance of the active site gorge in AChE, is absent in BChE (Harel et al., 1992). According to Rosenberry (1975), AChE is more sensitive to the size of the acyl group than to the alcohol moiety (whether charged or neutral) of the substrate, while for BChE the opposite is observed. Both are inhibited by 50 µM of physostigmine (eserine), which is a condition that affords to discriminate cholinesterases (ChEs) from other esterases (Augustinsson, 1963).
The class of AChEs is more homogeneous in terms of their primary structure than the class of BChEs (Rosenberry, 1975). Despite of these differences, the amino acid sequence identity between AChE and BChE from vertebrates ranges from 53 to 60%, even in evolutionarily distant species (Chatonnet & Lockridge, 1989; Taylor, 1991). In addition, a study promoted the replacement of only two amino acids by site-directed mutagenesis in AChE for it to develop BChE activity (Harel et al., 1992). Both enzymes present the active site within a deep and narrow gorge, approximately in the middle of its globular structure, which apparently could disturb the substrate traffic. However, in fact this structure follows a rational organization which entraps substrate and transports it to the active site through the arrangement of amino acids lining the gorge. All this occurs very efficiently (Quinn, 1987; Tõugo, 2001).
2.2. Acetylcholinesterases of invertebrates
Only globular forms of AChE are present in invertebrates (Massoulié et al., 1993; Talesa et al., 1993, 1996; Pezzementi et al., 1989; Sanders et al., 1996). Such enzymes consist of monomers (G1), dimers (G2) and tetramers (G4) of catalytic subunits, without the asymmetric collagenic-tailed forms typical of vertebrates. Generally, most AChEs described in invertebrates display less defined substrate specificity and a marked variability in the kinetic behavior compared to vertebrates (Talesa et al., 1994). In particular, a dimeric AChE linked to the cell membrane by a phosphatidylinositol (PtdIns) anchor is always expressed in invertebrates. Such an enzyme is thought to fulfil a key role in cholinergic transmission, while the function of similar AChE forms is so far unknown in vertebrates (Romani et al., 2006).
In invertebrates, the genetics of AChEs differ in the various phyla and sometimes within the same phylum: the AChE subunits are encoded by one, two or several genes (Talesa et al., 1999, 2002; Romani et al., 2006), thus giving a complex polymorphism of the enzyme.
3. CHOLINESTERASE INIBITORS AND MECHANISM OF ENZYME INHIBITION
A wide range of contaminants including organophosphorus and carbamates pesticides, heavy metals, and polycyclic aromatic hydrocarbons may affect ChE (Jebali et al., 2006; Elumalai et al., 2007; Bonacci et al., 2008). The best known inhibitors of ChE are those belonging to the carbamate and organophosphate classes. Organophosphorus esters (OPs) and carbamates form two important classes of agrochemicals. Most of the commercially relevant bioactives OPs have sulfur direct attached to the central pentavalent phosphorus.
Organophosphorus pesticides have the following general chemical structure:
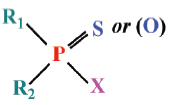
where the R-groups may be alkoxy, alkyl, aryl, or amide. The X-group is generally a carboxylating, cyanide, thiocyanate, phosphate, halide, phenoxy, or thiophenoxy group Carbamate insecticides have the following structures:
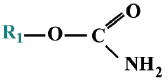
Carbamates and organophosphates generally have low environmental persistence, especially when compared with organochlorine pesticides. Their degradation is often accelerated by an increase in temperature or alkalinity (Kanazawajun 1975; Solé et al., 2000). Although organophosphorus and carbamate pesticides are chemically different, exposure to either of these groups of pesticides usually results in the inhibition of cholinesterase enzyme (ChE) activity.
Acetylcholine (ACh), the neurotransmitter secreted by cholinergic postganglionic neurons, allows for transmission of nerve impulses across the synapse. Acetylcholinesterase (AChE; Enzyme Commission Number 3.1.1.7) plays an important role in neurotransmission in both vertebrates and invertebrates, being responsible for the hydrolysis of acetylcholine into choline and acetic acid at the cholinergic synapses and neuromuscular junctions (Penã-Lopis et al., 2003). Acetylcholinesterase or true cholinesterase is found in high concentration in the nervous system. Pseudocholinesterase (Butyrylcholinesterase (BChE; peopionylcholinesterase (PChE)) is found in muscle at variable proportions of both aquatic vertebrate and invertebrate; although its physiological function has not been well defined, it is useful as an indicator of organophosphate and carbamate exposure (Massoulié et al., 1993).
The figure 2 shows schematically the mechanisms of action of organophosphorus and carbamates on acetylcholinesterase. The molecular activity of anticholinesterase OPs associated with the inhibition mechanism consists in a nucleophilic attack of the serinic oxygen of cholinesterase active site to the phosphorus atom of OPs. Cleavage of the acidic OP leaving group enables formation of a covalent P-O (serine) bond, thus inhibiting enzymatic hydrolysis of the natural substrate acetylcholinesterase, which would proceed via a reaction between serine OH and the electrophilic carbonylcarbon of the neurotransmitter. Phosphorothionates (ester containing the thiol group P=S) are less effective as AChE inhibitors than their oxon metabolites (with P=S being replaced by O=P), which are formed in vivo by cytochrome P-450 oxidation (Mastrantonio et al., 2008):
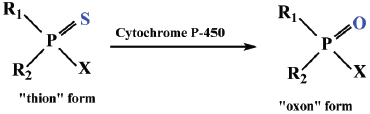
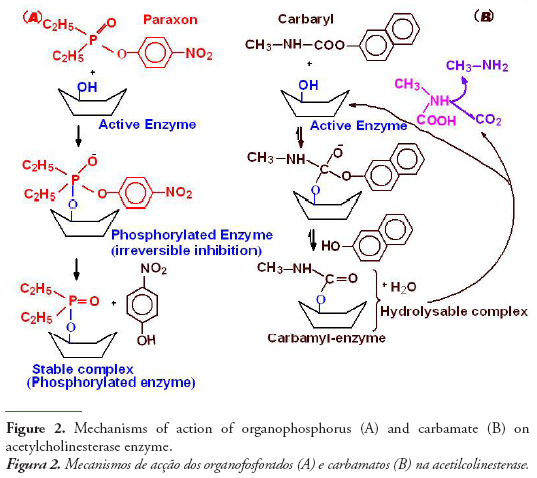
Phosphorothionates are less reactive to hydrolysis and more lipophilic than phosphates, which makes them more efficient in reaching critical endogenous sites in sufficiently effective concentrations, with cytochrome P-450 oxidation to oxon metabolites forming a major toxification step in vivo. Organophosphorus chemicals phosphorylate acetylcholinesterase in an irreversible reaction that inhibits the activity of cholinesterase to hydrolyze the neurotransmitter at the nerve synapse. The accumulation of acetylcholine results in a continuous nerve firing and eventual failure of nerve impulse propagation. Respiratory paralysis is generally the immediate cause of death (Massoulié et al., 1993).
One of the main targets of carbamates is acetylcholinesterase activity, as organophosphorus, but less reactive (See Fig. 2), since in this case the complex carbamyl-enzyme is hydrolysable with release of CO2, methylamine and the regenerated enzyme. The same kinds of troubles neurohormonal as organophosphorus by O’Brien (1976), but the degree of inhibition is reduced in proportion to rate of the enzyme regeneration. In addition to anticholinesterase pesticides, some organic and metallic compounds such as polyaromatic hydrocarbons and metals have the potential to inhibit cholinesterase enzyme (ChE) activity, but the mechanisms of inhibition is not fully known (Jebali et al., 2006). Some in vivo studies have reported inhibitory effects (but sometimes activating effects) of metals on fish brain AChE activity (Jebali et al., 2006), but the concentrations used in these tests are usually far removed from the concentrations measured in situ. As with all enzymes, cholinesterases conformation can be modified by the presence of metals, so there are no specific effects of these types of pollutants on cholinesterases (Bocquené et al., 1997).
4. CHOLINESTERASE AS A BIOMARKER OF NEUROTOXICITY: UTILITY IN THE ASSESSMENT OF AQUATIC ENVIRONMENT
ChE activity has been widely studied and employed as a biomarker in aquatic invertebrate and vertebrate species to detect exposure to chemicals in natural ecosystem (Dellai et al., 2001; Roméo et al., 2003; Lavado et al., 2006). The potential use of ChE activity in sentinel species for monitoring both environmental quality and the health of organisms inhabiting polluted ecosystems has received increasing attention during the recent years. In fact, we measured the cholinesterase activity in organs from endemic species collected from different sites in Tunisia. Indeed, Cyprinus carpio is widely used as sentinel organism in biomonitoring programs and toxicity bioassays (Van der Oost et al., 1998; Chuiko, 2000; De la Torre et al., 2002). We measured the AChE and BChE in brain and muscle of common carp Cyprinus carpio sampled from three Tunisian dam lakes submitted to various adverse environmental conditions. We found a high inhibition of AChE activity in both organs, since the BChE was significantly reduced only in muscle (Tlili et al., 2010a). Ozmen et al. (2007) showed that there is a strong relationship between AChE inhibition in the brain of C. carpio collected from Sariyar Dam Lake (Turkey) and the organochlorine pesticides and their residues in water, sediments, and C. carpio adipose tissues. In other in field investigations using AChE as neurotoxicity biomarker, Lavado et al. (2006) reported that AChE was strongly inhibited in the muscle of C. carpio sampled from some stations of Ebro River (Spain) highly polluted by organophosphorous, carbamates, and heavy metals. The measurement of cholinesterase in the brain or muscles, involves the sacrifice of the animals to provide information on both exposure and toxic effects on animals. The serum cholinesterase determination, achievable by non-invasive methods, does not, in general reliably assess the toxic effects associated with exposure (Bocquené et al., 1997). Regarding the tissue distribution, we found in the following rank of AChE activity in Solea solea fish: brain > gills> kidney>liver (Table 1) (Jebali et al., 2012). The reasons for these differences could be due to innervations levels between the different tissues. Accordingly, different tissues did not respond in the same way to both exposure and effects of pollutants. S. solea is a sentinel fish for environmental studies because, as a flatfish, it lives in a restricted range. Thus, if there is evidence for exposure of S. solea to chemical contaminants, it can be used to determine where the exposure occurred (Ribecco et al. 2012). In recent years, it has also been selected as sentinel infield pollution monitoring and laboratory toxicity experiments (Davoodi & Claireaux 2007; Trisciani et al. 2011; Palermo et al. 2012). Recent field study, showed a significant inhibition of brain AChE in S. solea fish collected from polluted sites, whereas small changes were observed in gills, liver and kidney AChE (Table 1) (Jebali et al., 2012). This inhibition is due to the polluted sites. In fact, Fathallah et al. (2012) clearly showed that the seawater of Monastir bay contained high concentrations of metals expressed in ppm: Cd = 7.24, Pb = 19.5, Cu = 15.7, Ni = 3.8, Zn = 48.7, Hg = 18.1. Indeed, a geochemical study at Monastir bay by Dahmane (2000) showed that the levels of heavy metals such as copper (> 150 ppm ), lead (> 150 ppm) , zinc (<75 ppm) , cadmium (50 - 100 ppm) and manganese (<70 ppm) in sea water at the site of Khniss are higher than those recorded by Goldberg et al. (1970) and Martin (1976) in the unpolluted sea and suggested that the Khniss site is highly polluted by heavy metals originating from contributions by the drain Khniss, fishing activities and it receives several domestic wastes from the surrounding areas and industrial activities in the region site (Kessabi et al., 2013; Zrafi et al., 2013). In Khniss, the levels of aromatic hydrocarbons are ranging from 1-14 mg/l (7-33 % of total hydrocarbons) in seawater and from 2280-7700 µg/g (5-9% of total hydrocarbons) in sediments (Zrafi et al., 2013). Similarly, it has been shown by a chemical study in surface sediments at Khniss and Sayada that concentrations of cadmium (1-6 ppm), zinc (75-100 ppm), copper (15-30 ppm) and lead (45-100 ppm) are very high compared to levels reported in other Tunisian sites (Gulf of Tunis) (Sahnoun, 2000; Rezgui, 2007). Teboulba is influenced by the treated and none treated municipal wastewater from limitroph agglomerations and fishing harbor (Jebali et al., 2011). At this site, PAH levels in seawater range from 4-22 mg/l (3-31% of total hydrocarbons) in sea water and from 93-1050 mg/g in superficial sediments. This charge of PAHs would be petroleum from the fishing and port.
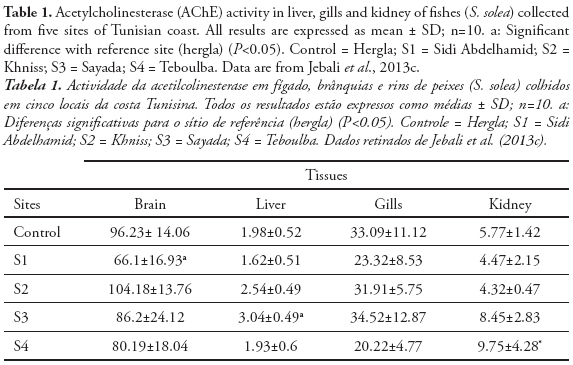
The levels of total hydrocarbons in sediments of Sidi Abdelhamid vary between 30 and 70 mg/kg. This slight contamination is caused mainly by fishing boats and pleasure craft Sabbagh, 2011). Solé et al. (2012) reported that S. senegalensis AChE was dominant in brain (53–65 %), followed by kidney (11– 13 %), liver (4–7 %) and gills (4 %). Koenig et al. (2013) showed that S. solea AChE was inhibited in brain after exposure to chlorpyriphos. These results could indicate the highest sensitivity of the brain in comparison with the other organs. The differences in the sensitivity of the tissues may be related to the pollutants’ difficulty in reaching the sites where AChE is located: e.g. it may be easier to reach the synapses in the brain than the neuromuscular junctions in the gills. So far, these findings point out the convenience of focusing on fish brain during future monitoring.
The use of ChE activity as an environmental biomarker requires a careful characterization of the enzymes present in a given species and tissue to minimize possible erroneous interpretation of the results (Vioque-Fernández et al., 2007). ChE activities have been investigated in tissues of the cockle Cerastoderma glaucum. The basal levels of enzyme activities, substrate specificity and tissue distribution were characterized and the kinetic response under natural conditions was evaluated using a caging approach. The results of the preliminary screening of ChE activity in whole animal, rest of animal (adductor and retractor muscles, mantle and foot), visceral mass and gills of Cerastoderma glaucum (Table 2) show that the highest ChE activity was obtained using ASCh and PSCh, whereas the hydrolysis of BSCh was noticeably low (Jebali et al., 2011).
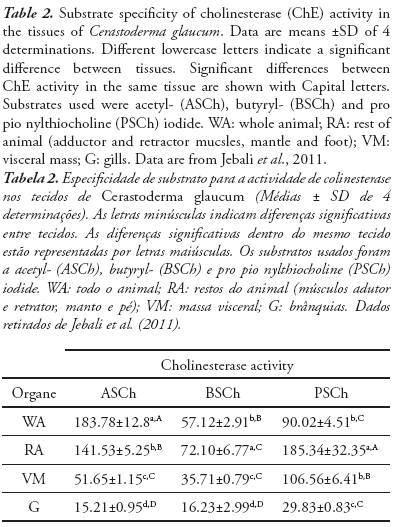
In view of future national biomonitoring programmes, we illustrate the different sensitivity and kinetic response of AChE activity after caging exposure of C. glaucum at fishing harbour. In fact, we know that Over 450 ships of different size are based in this harbour (Jebali et al., 2013a), thus receiving contaminants from ship traffic and waste from fish industry, that represent a key pollutant source for the central Tunisian littoral (Banni et al., 2005; Jebali et al., 2007; 2011). Therefore, the pollutants responsible for the inhibition of AChE are the Polycyclic aromatic hydrocarbons (PAH) and other pro-oxidant chemicals, present also in Téboulba fishing harbor (Jebali et al., 2013a). Cerastoderma glaucum caged at Teboulba fishing harbour for 4 week experienced a critical alteration in gill AChE activity compared with the tissue activity of the rest of the animal (Table 3). The control Cerastoderma glaucum was collected from Kuriat. This site is characterized by the absence of any source of pollution (Jebali et al., 2011; Ben-Khedher et al., 2013). In the gills, AChE activity decreased in a time-dependent pattern, losing approx. 15 and 68% activity after 2 and 4 week of transplantation, respectively, whereas in muscle tissue (rest of animal) the loss of AChE was approximately 24% at the end of the 4 week caging period (Jebali et al., 2011) Consequently, different profile responses of AChE activity were noted and the gill seems to be more sensitive to the effect of harbour pollutants than the muscle. In fact, few data exist about the measurement of ChE activity in Cerastoderma glaucum (Machreki-Ajmi et al., 2008), but this does not prevent the use of this species as an interesting bioindicator in other ecotoxicological studies worldwide (Matozzo et al., 2007; González-Wangüermert et al., 2009; Borówka et al., 2012).
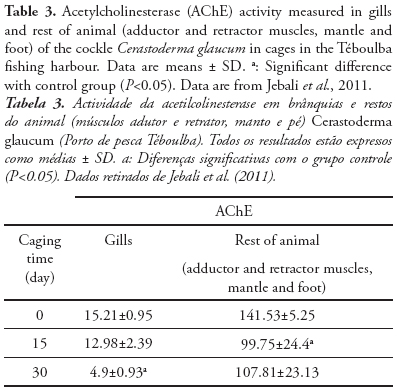
AChE can change significantly in Tunisian bivalve species in response to different stress conditions. In Donax trunculus, the enzymatic activity can change seasonally, mostly according to reproduction cycles, water temperature and food availability and pollution level (Tlili et al., 2010b). The marked inhibition observed in AChE activities in bivalves from polluted sites (Gulf of Tunis, Tunisia), during the warm period could be explained by the negative effect of temperature as recently reported in other marine species crabs (Ben Khedher et al., 2013). Moreover, the effect of temperature on AChE activity in D. trunculus from the polluted site appeared to act synergistically with the effect of anthropogenic inputs by comparison with the reference site (Tlili et al., 2010b). In order to use AChE inhibition as a pollution biomarker, it is necessary that the change due to contamination exceeds the natural variability (Bocquené et al., 1997). Indeed, in the case of in situ biomonitoring, it is difficult to separate the contribution of abiotic factors and the chemical pollutants in the response of the AChE activity. However, the contribution of abiotic factors is low compared with the effect of chemical contaminants. Also, the use of control can clearly show the effects of chemical pollutants on AChE activity. Ben Khedher et al. (2013) showed the inhibition of AChE activity in Carcinus maenas collected from four contaminated sites from Bizerta lagoon and from Kuriat as control site in February and July and those in both organs (gills and digestive gland) (Fig 3). In fact, this inhibition is due to the presence of contaminants (metals and hydrocarbons) in water and sediments in these sites (Table 4). Positive correlations were recorded between the AChE in gills and chemical parameters in water (Cd, r = 0.925, p<0.01; Cu, r = 0.821, p<0.01; Mn, r = 0.895, p<0.01; Ni, r = 0.846, p<0.01) and in sediment (Cd, r = 0.688, p<0.05; Fe, r = 0.653, p<0.05; Pb, r = 0.912, p<0.01; Mn, r = 0.685, p<0.05; Ni, r = 0.688, p<0.05; hydrocarbons, r = 0.730, p<0.05).
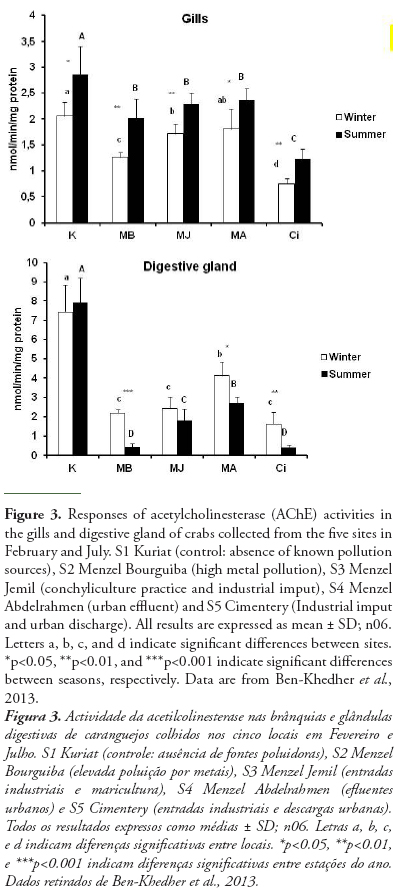
In general, 20% or greater depression in AChE activity in several species invertebrates can be an indicator of exposure to pesticides (Day and Scott 1990). Moreover, we investigated the effect of OP (chlorpyrifos-ethyl: CPF) at sublethal concentrations and after 3 days of exposure under experimental conditions on AChE and BuChE in target tissues of crab Carcinus maenas (Ghedira et al., 2011). This work demonstrated that for both gills and hepatopancreas, the AChE activity was higher than BuChE activity. This result indicated that enzyme activity measured in two tissues under our experimental conditions showed a preference for acetylthiocholine as substrate over butyrythiocholine. The results showed also that the exposure of C. maenas to OP led to the inhibition of ChE activities (AChE and BuChE).
AChE in bivalve from the Tunisian coast can also change in response to pollutant exposure. Metals such as Ni, under acute exposure, are able to decrease AChE activity in dose-dependant in M. galloprovincialis (Attig et al., 2010). Interestingly, AChE activity inhibition was correlated with the Ni uptake in the digestive gland of mussels exposed to both the tested concentrations (2.5 and 13 mM Ni). In another study, R. decussatus clams exposed continuously for 14 day to treated municipal effluents had seriously altered the AChE in gills and digestive gland. Interestingly, AChE activity inhibition was correlated with the Zn, Cu and Cd accumulation in both organs (digestive gland and gills) of treated clams (Kamel et al., 2012).
Furthermore, pesticides, carbamates and heavy metals are known by their capacity to inhibit in vitro or in vivo, AChE activity (Galloway et al., 2004; Banni et al., 2005), especially in fish (Monserrat et al., 2002. Sturn et al., 2000; Kirby et al., 2000). In fact, de la Torre et al. (2002) noted that the inhibition of fish brain AChE can be detected soon after the beginning of “in field” exposure to OP. AChE inhibition was observed in the fishes Seriola dumerilli exposed under laboratory conditions to Cd (Jebali et al., 2006). Indeed, the neurotoxicity effect of Cd illustrated in many in-field and in-vivo studies using aquatic organisms as bioindicators (Roméo et al., 2006). Jebali et al. (2013b) investigated the effects of Ni and CPF on AChE activity in sea bass (Dicentrarchus labrax) following 1, 3 and 7 days exposure (Table 5). CPF have a higher significant repression on brain AChE at day1 and then its return to the physiological activity at day 3 and 7. The lack of AChE inhibition after 3 and 7 days in D. labrax seen in this work would indicate the total detoxification of CPF after this period and the recuperation of AChE activity, probably due to the novo synthesis (Table 5). In the contrary of no effect of Ni on AChE activity, the mixture Ni and CPF, highly enhanced this activity in the late exposure time (day 3 and 7), consequently displaying neurotoxicity to the sea bass, which was inconsistent with the previous studies which show that metals or metals associated with pesticide may exert toxicity on the AChE activity (Scheil & Köhler, 2009; Wang & Wang, 2009).
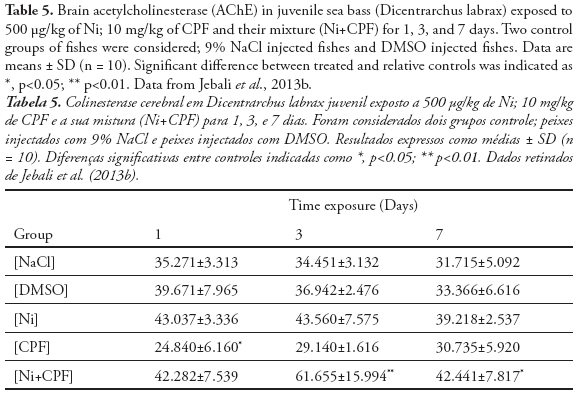
5. CONCLUSION
The use of ChE activity in species cultivated for human consumption such as bivalves and fishes is of great importance for the assessment of the aquatic environment contamination. The results presented and discussed here indicate that different environmental factors, like the exposure to different classes of contaminants, can cause substantial changes in cholinesterase activity. The characterization and the evaluation of the AChE activity of these aquatic organisms may be useful for biomonitoring of other natural ecosystems.
ACKNOWLEDGMENTS
Studies mentioned in this review were supported by the fund from the Ministry of Scientific Research and Technology, University of Sousse, Tunisia (Research Unit of Biochemistry and Environmental Toxicology UR 04AGR05), and “Institution de la Recherche et de l’Enseignement Supérieur Agricole, (IRESA; Tunisia)”. We are most grateful to Pr. Mohamed Ben Khedher for extensive English revision to improve the quality of the review.
REFERENCES
Attig, H.; Dagnino, A.; Negri, A.; Jebali, J.; Boussetta, H.; Viarengo, A.; Dondero, F.; Banni, M. (2010) - Uptake and biochemical responses of mussels Mytilus galloprovincialis exposed to sublethal nickel concentrations. Ecotoxicology and Environmental Safety 73(7):1712-1719, doi: 10.1016/j.ecoenv.2010.08.007. [ Links ]
Augustinsson, K.B. (1963) - Cholinesterase and anticholinesterase agents. In: G.B. Koelle, (org),. Handbook of Experimental Pharmacology, pp.89-128, Springer-Verlag, Berlin, Germany. ISBN: 978-3540029885. [ Links ]
Banni, M.; Jebali, J.; Daubeze, M.; Clerandau, C.; Guerbej, H.; Narbonne, J.F.; Boussetta, H. (2005) - Monitoring pollution in Tunisian coasts: application of a classification scale based on biochemical markers. Biomarkers, 10(2-3):105-116. doi: 10.1080/13547500500107497. [ Links ]
Ben-Khedher, S.; Jebali, J.; Kamel, N.; Banni, M.; Rameh, M.; Jrad, A.; Boussetta, H. (2013) - Biochemical effects in crabs (Carcinus maenas) and contamination levels in the Bizerta Lagoon: an integrated approach in biomonitoring of marine complex pollution. Environmental Science and Pollution Research, 20(4):2616-2631. doi: 10.1007/s11356-012-1156-x. [ Links ]
Bocquen., G.; Galgani, F.; Walker, C.H. (1997) – Les cholinest.rases, biomarqueurs de neurotoxicit.. In: L. Lagadig, T. Caquet, J.C. Amiard & F. Ramade (eds.), Biomarqueurs en écotoxicologie. Aspect fondamentaux, pp.209-240, Masson, Paris, France.ISBN: 2225830533.
Bonacci, S.; Corsi, I.; Focardi S. (2008) - Cholinesterase activities in the scallop Pecten jacobaeus: Characterization and effects of exposure to aquatic contaminants. Science Of The Total Environment, 392(1):99-109. doi: 10.1016/j.scitotenv.2007.11.029. [ Links ]
Borówka, R.K.; Strobel, W.; Hałas, S. (2012) - Stable isotope composition of subfossil Cerastoderma glaucum shells from the Szczecin Bay brackish deposits and its palaeogeographical implications (South Baltic Coast, Poland). Quaternary Research 77(2):245-250, doi: 10.1016/j.yqres.2012.01.001 [ Links ]
Chatonnet, A.; Lockridge, O. (1989) - Comparison of butyrylcholinesterase and acetylcholinesterase. Biochemical, 260(3):625-634. Available at http://www.ncbi.nlm.nih.gov/pmc/articles/PMC1138724/ [ Links ]
Chuiko, G.M. (2000) - Comparative study of acetylcholinesterase and butyrylcholinesterase in brain and serum of several freshwater fish: Specific activities and in vitro inhibition by DDVP, an organophosphorus pesticide. Comparative Biochemistry and Physiology Part C, 127(3):233–242. doi: 10.1016/S0742-8413(00)00150-X.
Çokugras, A.N. (2003) - Butyrylcholinesterase: Structure and Physiological Importance. Turkish Journal of Biochemistry, 28(2):54-61. Available at http://www.turkjbiochem.com/2003/054_061.pdf. [ Links ]
Davoodi, F.; Claireaux, G. (2007) - Effects of exposure to petroleum hydrocarbons upon the metabolism of the common sole Solea solea. Marine Pollution Bulletin, 54(7):928-934. doi: 10.1016/j.marpolbul.2007.03.004 [ Links ]
Day, K.E.; Scott, I.M. (1990) - Use of acetylcholinesterase activity to detect sublethal toxicity in stream invertebrates exposed to low concentrations of organophosphate insecticides. Aquatic Toxicology, 18(2-3):201-224. doi: 10.1016/0166-445X(90)90021-G [ Links ]
de la Torre, F.R.; Ferrari, L.; Salibian, A. (2002) - Freshwater pollution biomarker: Response of brain acetylcholinesterase activity in two fish species. Comparative Biochemistry and Physiology Part C 131 (3): 271-280. Available at http://www.sciencedirect.com/science/article/pii/S1532045602000145. [ Links ]
Dellali, M.; Gnassia-barelli, M.; Romeo, M.; Aissa, P.; (2001) - The use of acetylcholinesterase activity in Ruditapes decussatus and Mitillus galloprovincialis in the biomonitoring of Bizerta lagoon. Comparative Biochemistry and Physiology, 130(2):227-235. doi: 10.1016/S1532-0456(02)00014-5 [ Links ]
Elumalai, E.; Antunes, C.; Guilhermino. L. (2007) - Enzymatic biomarkers in the crab Carcinus maenas from the Minho River estuary (NM Portugal) exposed to zinc and mercury. Chemosphere, 66(7):1249-1255. doi: 10.1016/j.chemosphere.2006.07.030 [ Links ]
Fathallah, S.; Medhioub, M.N.; Medhioub, A.; Kraiem, M.M. (2011) - Ruditapes decussatus embryo–larval toxicity bioassay for assessment of Tunisian coastal water contamination. Environmental Chemistry and Ecotoxicology, 3(11):277-285. Available at http://academicjournals.org/article/article1380009080_Fathallah_et_al.pdf.
Forget, J.; Bocquené, G. (1999) - Partial purification and enzymatic characterization of acetylcholinesterase from the intertidal marine copepod Tigriopus brevicornis. Comparative Biochemistry and Physiology Part B, 123(4):345–350. doi: 10.1016/S0305-0491(99)00073-5
Galloway, T.S.; Brown, R.J.; Browne, M.A.; Dissanayake, A.; Lowe, D.; Jones, MB.; Depledge, M.H. (2004) - A multibiomarker approach to ecosystem management. Environmental Science and Technology, 38:1723-1731. [ Links ]
Ghedira, J.; Jebali, J.; Banni, M.; Chouba, L.; Boussetta, H.; López-Barea, J.; Alhama, J. (2011) - Use of oxidative stress biomarkers in Carcinus maenas to assess littoral zone contamination in Tunisia. Aquatic Biology, 14:87-98. doi:10.3354/ab00377 [ Links ]
Goldberg, E.D.; Broecker, W.S. (1970) - Marine chemistry. In National Academy of Sciences. National Reseach Council 137-146. [ Links ]
González-Wangüemert, M.; Cánovas, F.; Marcos, C.; Pérez-Ruzafa, A. (2009) - Phosphoglucose isomerase variability of Cerastoderma glaucum as a model for testing the influence of environmental conditions and dispersal patterns through quantitative ecology approaches. Biochemical Systematics and Ecology, 37(4):325–333. doi: 10.1016/j.bse.2009.05.013
Harel, M.; Sussman, J.L.; Krejci, E.; Bon, S.; Chanal, P.; Massouli., J.; Silman, I. (1992) - Conversion of acetylcholinesterase to butyrylcholinesterase: Modeling and mutagenesis. Proceedings of the National Academy of Sciences of the United States of America, 89(22):10827-10831. Available at http://www.pnas.org/content/89/22/10827.full.pdf [ Links ]
Helmut, S.; Braunbeck, T. (1998) - Cellular response profile to chemical stress. In: Gerrit Schüürmann &Bernd Markert (eds.), Ecotoxicology: Ecological Fundamentals, Chemical Exposure, and Biological Effects, pp.521-569, Wiley, New York, U.S.A. ISBN: 0471176443 [ Links ]
Jebali, J.; Banni, M.; Guerbej, H.; Almeida, E.A.; Bannaoui, A.; Boussetta, H. (2006) - Effects of malathion and cadmium on acetylcholinesterase activity and metallothionein levels in the fish Seriola dumerilli. Fish Physiology Biochemistry, 32(1):93-98. doi: 10.1007/s10695-006-0041-2. [ Links ]
Jebali, J.; Banni, M.; DeAlmeida, E.A.; Boussetta, H. (2007) - Oxidative DNA damage levels and catalase activity in the clam Ruditapes decussatus as pollution biomarkers of Tunisian marine environment. Environmental Monitoring Assessment, 124:195-200. doi: 10.1007/s10661-006-9217-6. [ Links ]
Jebali, J.; Ben-Khedher, S.; Kamel, N.; Ghedira, J.; Bouraoui, Z.; Boussetta, H. (2011) - Characterization and evaluation of cholinesterase activity in the cockle Cerastoderma glaucum. Aquatic Biology, 13:243–250. doi:10.3354/ab00365
Jebali, J.; Sabbagh, M.; Banni, M.; Kamel, N.; Ben-Khedher, S.; M’hamdi, N.; Boussetta, H. (2013c) - Multiple biomarkers of pollution effects in Solea solea fish on the Tunisia coastline. Environmental Science and Pollution Research, 20(6):3812-3821. doi: 10.1007/s11356-012-1321-2.
Jebali, J.; Chicano-Gálvez, E.; Banni, M.; Guerbej, H.; Boussetta, H.; López-Barea, J.; Alhama, J. (2013a) – Biochemical responses in sea bream (Sparus aurata) caged in-field or exposed to benzo (a) pyrene and paraquat. Characterization of glutathione S-transferases. Ecotoxicology and Environmental Safety, 88:169–177. doi: 10.1016/j.ecoenv.2012.11.013.
Jebali, J.; Banni, M.; Ben-Khedher, S.; Guerbej, H.; Boussetta, H. (2013b) - Biochemical Response of Sea bass Dichentrarchus labrax Experimentally Exposed to Nickel and Chlorpyriphos. Biomarkers in Drug Development, 2(1): online doi: 10.4172/jbdd.1000101. [ Links ]
Johnson, G.; Moore, S.W. (2000) - Cholinesterases modulate cell adhesion in human neuroblastoma cells in vitro. International Journal of Developmental Neuroscience, 18(8):781–790. doi: 10.1016/S0736-5748(00)00049-6
Kamel, N.; Jebali, J.; Banni, M.; Ben-Khedher, S.; Chouba, L.; Boussetta, H. (2012) -Biochemical Responses and metals levels in Ruditapes decussatus after exposure to treated municipal effluents. Ecotoxicology and Environmental Safety, 82:40-46. doi: 10.1016/j.ecoenv.2012.05.008. [ Links ]
Kanazawajun, J. (1975) - Uptake and Excretion of Organophosphorus and Carbamate Insecticides by Fresh Water Fish, Motsugo, Pseudorasbora parva. Bulletin of Environmental Contamination & Toxicology, 14(3):346-352. Available at http://www.ncbi.nlm.nih.gov/pubmed/1174751. [ Links ]
Kessabi, K.; Annabi, A.; Ibn Hadj Hassin, A.; Bazin, I.; Mnif, W.; Said, K.; Messaoudi, I. (2013) - Possible chemical causes of skeletal deformities in natural populations of Aphanius fasciatus collected from the Tunisian coast. Chemosphere, 90(11):2683–2689. doi: 10.1016/j.chemosphere.2012.11.047.
Kirby, M.F.; Morris, S.; Hurst, M.; Kirby, S.J.; Neall, P.; Tylor, T. (2000) - The use of cholinesterase activity in flounder (Platichthys flesus) muscle tissue as a biomarker of neurotoxic contamination in UK estuaries. Marine Pollution Bulletin, 40(9):780–791. doi: 10.1016/S0025-326X(00)00069-2
Koenig, S.; Guillén, K.; Solé, M. (2013) - Comparative xenobiotic metabolism capacities and pesticide sensitivity in adults of Solea solea and Solea senegalensis. Comparative Biochemistry and Physiology Part C, 157(4):329–336. doi: 10.1016/j.cbpc.2013.03.002.
Kovarik, Z.; Bosak, A.; Sinko, G.; Latas, T. (2003) - Exploring the Active Sites of Cholinesterases by Inhibition with Bambuterol and Haloxon. Croatica Chemica Acta, 76(1):63-67. Available at http://cat.inist.fr/?aModele=afficheN&cpsidt=14767748. [ Links ]
Lavado, R.; Ureña, R.; Martin-Skilton. R.; Torreblanca, A.; Del Ramo, J.. Raldúa, D.; Porte, C. (2006) - The combined use of chemical and biochemical markers to assess water quality along the Ebro River. Environmental Pollution, 139(2):330–339. Available at http://www.ncbi.nlm.nih.gov/pubmed/16040174.
Livingstone, D.R. (1998) - The fate of organic xenobiotics in aquatic ecosystems: quantitative and qualitative differences in biotransformation by invertebrates and fish. Comparative Biochemistry and Physiology Part A, 120(1):43-49. Available at http://www.ncbi.nlm.nih.gov/pubmed/9773498. [ Links ]
Lopez-Carillo, L.; Lopez-Cervantes, M. (1993) - Effect of exposure to organophosphate pesticides on serum cholinesterase levels. Archives of environmental health, 48(5):359-363. Available at http://www.ncbi.nlm.nih.gov/pubmed/8215602. [ Links ]
Machreki-Ajmi, M.; Ketata, I.; Ladhar-Chaabouni, R.; Hamza-Chaffai, A. (2008) - The effect of in situ cadmium contamination on some biomarkers in Cerastoderma glaucum. Ecotoxicology, 17(1):1-11. Available at http://www.ncbi.nlm.nih.gov/pubmed/18060580. [ Links ]
Massoulié, J.; Pezzementi, L.; Bon, S.; Krejci, E.; Vallette, F.M.; (1993) - Molecular and cell biology of cholinesterases. Progress in Neurobiology, 41(1):31-91. Available at http://www.ncbi.nlm.nih.gov/pubmed/8321908. [ Links ]
Mastrantonio, G.; Mack, H.G.; Della Védova, C.O. (2008) - Interpretation of the mechanism of acetylcholinesterase inhibition ability by organophosphorus compounds through a new conformational descriptor. An experimental and theoretical study. Molecular Model, 14(9):813-821. doi: 10.1007/s00894-008-0321-0. [ Links ]
Matozzo, V.; Marin, M.G. (2007) - First evidence of altered vitellogenin-like protein levels in clam Tapes philippinarum and in cockle Cerastoderma glaucum from the Lagoon of Venice. Marine Pollution Bulletin, 55(10-12): 494-504. Available at http://www.ncbi.nlm.nih.gov/pubmed/17936854. [ Links ]
Monserrat, J.M.; Bianchini, A.; Bainy, A.C.D. (2002) - Kinetic and toxicological characteristics of acetylcholinesterase from the gills of oysters (Crassostrea rhizophorae) and other aquatic species. Marine Environmental Reseach, 54(3-5): 781–785. Available at http://www.ncbi.nlm.nih.gov/pubmed/12408650.
Monserrat, J.M.; Martínez, P.E.; Geracitano, L.; Amado, L.L.; Gaspar Martins, C.M.; Leães Pinho, G.L.; Chaves, I.S.; Ferreira-Cravo, M.; Ventura-Lima, J.; Bianchini, A.; (2007) - Pollution biomarkers in estuarine animals: critical review and new perspectives. Comparative Biochemistry and Physiology Part C, 146(1-2):221-234. Available at http://www.ncbi.nlm.nih.gov/pubmed/17045848. [ Links ]
Nicolet, Y.; Lockridge, O.; Masson, P.; Fontecilla-Camps, J.C.; Nachon, F. (2003) - Crystal Structure of Human Butyrylcholinesterase and of Its Complexes with Substrate and Products. Biological Chemistry, 278(42): 41141-41147. Available at http://www.ncbi.nlm.nih.gov/pubmed/12869558. [ Links ]
O’Brien, R.D. (1976) - Acetylcholinesterase and its inhibition. In: C.F. Wilkinson (ed.), Insecticide Biochemistry and Physiology, pp.271-296, Springer Science + Business Media, New York, NY, U.S.A. ISBN: 978-1489922144. [ Links ]
Ozmen, M.; Ayas, Z.; Gungurdu, A.; Ekmekci, G. F.; Yerli, S. (2007) - Ecotoxicological assessment of water pollution in Sariyar Dam Lake, Turkey. Ecotoxicology and Environmental Safety, 70(1):163–173. Available at http://www.ncbi.nlm.nih.gov/pubmed/17582495.
Palermo, FA.; Cocci, P.; Nabissi, M.; Polzonetti-Magni, A.; Mosconi, G. (2012) - Cortisol response to waterborne 4-nonylphenol exposure leads to increased brain POMC and HSP70 mRNA expressions and reduced total antioxidant capacity in juvenile sole (Solea solea). Comparative Biochemistry and Physiology Part C: Toxicology & Pharmacology, 156(3-4):135-139. doi: 10.1016/j.cbpc.2012.08.002. [ Links ]
Penã-Llopis, S.; Dolores Ferrando, M.; Penã, J.B. (2003) - Fish tolerance to organoohosphate- induced oxidative stress is dependent on the glutathione metabolism and enhanced by N-acetylcycteine. Aquatic Toxicology, 65(4):337-360. Available at http://www.ncbi.nlm.nih.gov/pubmed/14568351. [ Links ]
Pezzementi, L.; Chatonnet, A. (2010) - Evolution of cholinesterases in the animal kingdom. Chemico-Biological Interactions, 187(1-3):27–33. doi:10.1016/j.cbi.2010.03.043.
Pezzementi, L.; Nickson, H.C.; Dunn, R.C.; Bradley, R.G. (1989) - Molecular forms of acetylcholinesterase from the skeletal muscle of the ammocoete of the lamprey Petromyzon marinus. Comparative Biochemistry and Physiology Part B, 92(2):385–387. Available at http://www.ncbi.nlm.nih.gov/pubmed/2924545.
Quinn, D.M. (1987) - Acetylcholinesterase: enzyme structure, reaction dynamics, and virtual transition states. Chemical Reviews, 87(5):955-979. doi: 10.1021/cr00081a005. [ Links ]
Rezgui, A. (2007) - Contribution of geographic information systems for the determination of the impact of heavy metals on the surface sediments of coastal Monastir-Sayada. Diplôma of master. TUNIS II University, Tunisia. [ Links ]
Ribecco, C.; Hardiman, G.; Sášik, R.; Vittori, S.; Carnevali, O. (2012) - Teleost fish (Solea solea): a novel model for ecotoxicological assay of contaminated sediments. Aquatic Toxicology, 109:133–142. doi: 10.1016/j.aquatox.2011.12.002.
Romani, R.; Corsi, I.; Bonacci, S.; Focardi, S.; De Medio, G.E.; De Santis, A.; Incarnato, F.; Giovannini, E.; Rosi, G.; (2006) - Organophosphate-resistant forms of acetylcholinesterases in two scallops-the Antarctic Adamussium colbecki and the Mediterranean Pecten jacobaeus. Comparative Biochemistry and Physiology Part B, 145(2):188–196. Available at http://www.ncbi.nlm.nih.gov/pubmed/16931084.
Roméo, M.; Hoarau, P.; Garello, G.; Gnassia-Barelli, M.; Girard, J.P. (2003) - Mussel transplantation and biomarkers as useful tools for assessing water quality in the NW Mediterranean. Environmental Pollution, 122(3):369–378. doi: 10.1016/S0269-7491(02)00303-2
Rosenberry, T.L. (1975) - Catalysis by acetylcholinesterase: Evidence that the rate-limiting step for acylation with certain substrates precedes general acid-base catalysis Proceedings of the National Academy of Sciences of the United States of America, 72(10):3834-3838. Available at http://www.pnas.org/content/72/10/3834.full.pdf. [ Links ]
Sabbagh, M. (2011) - Study of biochemical biomarkers and biometrics in Solea solea: Validation of the use of some sites Tunisian coast. 60p., diplôma of master, Monastir University, Tunisia. Unpublished. [ Links ]
Sahnoun, O. (2000) - Geochemistry studies of heavy metals (Cu, Zn, Cd, Mn, Cr, Ni, Pb and Fe) in the sediments of Monastir bay. 132p., diplôma of master, Tunis II University,Tunisia. Unpublished. [ Links ]
Sanders, M.; Mathews, B.; Sutherland, D.; Soong, W.; Giles, H.; Pezzementi, L. (1996) -Biochemical and molecular characterization of acetylcholinesterase from the hagfish Myxine glutinosa. Comparative Biochemistry and Physiology Part B, 115(1):97-109. Available at http://www.ncbi.nlm.nih.gov/pubmed/8896335. [ Links ]
Scheil, V.; Köhler, H.R. (2009) - Influence of Nickel Chloride, Chlorpyrifos, and Imidacloprid in Combination with Different Temperatures on the Embryogenesis of the Zebrafish Danio rerio. Arch Environmental Contamination Toxicology, 56(2):238-243. doi: 10.1007/s00244-008-9192-8. [ Links ]
Silman, I.; Sussman, J.L. (2005) - Acetylcholinesterase: ‘classical’ and ‘non-classical’ functions and pharmacology. Current Opinion in Pharmacology, 5(3):293–302. Available at http://www.ncbi.nlm.nih.gov/pubmed/15907917.
Solé, M.; Porte, C.; Barcelo, D.; Albaiges, J. (2000) - Bivalves Residue Analysis for the Assessment of Coastal Pollution in the Ebro Delta (NW Mediterranean). Marine Pollution Bulletin, 40(9):746-753. doi: 10.1016/S0025-326X(00)00011-4 [ Links ]
Solé, M.; Vega, S.; Varó, I. (2012) - Characterization of type “B” esterases and hepatic CYP450 isoenzymes in Senegalese sole for their further application in monitoring studies. Ecotoxicology Environmental Safety, 78:72-79. doi: 10.1016/j.ecoenv.2011.11.013.
Soreq, H.; Zakut, H. (eds.) (1990) - Cholinesterase Genes Multileveled Regulation. 108p., S Karger Pub, Basel, Switzerland. ISBN 13: 9783805551373 [ Links ]
Sturm, A.; Wogram, J.; Segner, H.; Liess, M. (2000) - Different sensitivity to organophosphates of acetylcholinesterase and butyrylcholinesterase from three-spined stickleback (Gasterosteus aculeatus): application in biomonitoring. Environmental and Toxicology Chemosphere, 19:1607–15. Available at http://www.ufz.de/index.php?en=14784.
Sussman, J.L.; Harel, M.; Frolow, F.; Oefner, C.; Goldman, A.; Toker, L.; Silman, I.; (1991) - Atomic structure of acetylcholinesterase from Torpedo californica: a prototypic acetylcholine binding protein. Science, 253(5022): 872-879. doi: 10.1126/science.1678899 [ Links ]
Talesa, V.; Grauso, M.; Arpagaus, M.; Giovannini, E.; Romani, R.; Rosi, G. (1999) - Molecular cloning and expression of a full-length cDNA encoding acetylcholinesterase in optic lobes of Loligo opalescens: a new member of the cholinesterase family resistant to diisopropyl fluorophosphate. Neurochemical, 72(3):1250–1258. Available at http://www.ncbi.nlm.nih.gov/pubmed/10037498.
Talesa, V.; Principato, G.B.; Giovannini, E.; Grauso, M.; Rosi, G. (1993) - Evidence for a molecular polymorphism of Cholinesterase in Sepia officinalis (Cephalopoda: Decapoda). Comparative Biochemistry and Physiology Part B,106(3):557-562. doi: 10.1016/0305-0491(93)90132-O [ Links ]
Talesa, V.; Principato, G.B.; Giovannini, E.; Norton, S.J.; Rosi, G. (1994) - Presence of a solubile tetrameric (blood) and membrane-bound dimeric forms of cholinesterase in the mollusk Murex brandaris (Gastropoda: Neogastropoda). Experimental Zoology, 270:233–244. doi: 10.1002/jez.1402700302.
Talesa, V.; Romani, R.; Antognelli, C.; Giovannini, E.; Rosi, G.; (2002) - Different expressions of organophosphate resistant acetylcholinesterases in the bivalve mollusk Scapharca inaequivalvis living in three different habitats. Environmental Toxicology Chemistry, 21(1):102–108. Available at http://www.ncbi.nlm.nih.gov/pubmed/11804042.
Talesa, V.; Romani, R.; Rosi, G.; Giovannini, E. (1996) - Biochemical Characterization and Histochemical Localization in the Nervous System. European Journal of Biochemistry, 238(2):538-548. doi: 10.1111/j.1432-1033.1996.0538z.x [ Links ]
Taylor, P. (1991) - The cholinesterases. Biology Chemistry 266: 4025-4028. Available at http://www.jbc.org/content/266/7/4025.full.pdf+html. [ Links ]
Tlili, S.; Jebali, J.; Banni, M.; Haouas, Z.; Mlayah, A.; Helal, A.N.; Boussetta, H. (2010a) - Multimarker approach analysis in common carp Cyprinus carpio sampled from three freshwater sites. Environmental Monitoring and Assessment, 168:285-298. doi: 10.1007/s10661-009-1112-5. [ Links ]
Tlili, S.; Métais, I.; Boussetta, H.; Mouneyrac, C. (2010b) - Linking changes at sub-individual and population levels in Donax trunculus: Assessment of marine stress. Chemosphere, 81(6):692–700. doi: 10.1016/j.chemosphere.2010.07.064.
Tõugu, V.; (2001) - Acetylcholinesterase: mechanism of catalysis and inhibition. Current Medicinal Chemistry - Central Nervous System Agents, 1:155-170. doi:10.2174/1568015013358536. [ Links ]
Trisciani, A.; Corsi, I.; Della Torre, C.; Perra, G.; Focardi, S.; (2011) - Hepatic biotransformation genes and enzymes and PAH metabolites in bile of common sole (Solea solea, Linnaeus, 1758) from an oil contaminated site in the Mediterranean Sea: a field study. Marine Pollution Bulletin, 62(4):806–814. doi: 10.1016/j.marpolbul.2011.01.001.
Van der Oost, R.; Lopes, S.C.C.; Komen, H.; Satumalay, K. (1998) - Assessment of environmental quality and inland water pollution using biomarker responses in caged carp (Cyprinus carpio): Use of a bioactivation: Detoxication ratio as a Biotransformation Index (BTI). Marine Environmental Research, 46(1–5):315–319. doi: 10.1016/S0141-1136(97)00096-2
Vioque-Fernández, A.; Alves de Almeida, E.; Ballesteros, J.; García-Barrera, T.; Gómez-Ariza, J.L.; López-Barea, J. (2007) - Dõnana National Park survey using crayfish (Procambarus clarkii) as bioindicator: esterase inhibition and pollutant levels. Toxicology Letters, 168(3):260–268. Available at http://www.ncbi.nlm.nih.gov/pubmed/17157454.
Wang, M.H.; Wang, G.Z. (2009) - Biochemical response of the copepod Tigriopus japonicus mori experimentally exposed to cadmium. Arch Environmental Contamination Toxicology, 57(4):707–717. doi: 10.1007/s00244-009-9319-6.
Whittaker, M. (1980) - Plasma cholinesterase variants and the anaesthetist. Anaesthesia 35 (2): 174-97. Available at http://www.ncbi.nlm.nih.gov/pubmed/6992635. [ Links ]
Whittaker, M. (1986) - Cholinesterase. 134p., S Karger Pub, Basel, Switzerland. ISBN: 9783805542579 [ Links ]
Zrafi, I.; Bakhrouf, A.; Rouabhia, M.; Saidane-Mosbahi, D. (2013) - Aliphatic and aromatic biomarkers for petroleum hydrocarbon monitoring in Khniss Tunisian-Coast, (Mediterranean Sea) Procedia Environmental Sciences, 18: 211–220. doi: 10.1016/j.proenv.2013.04.027. al., 2011
*Submission: 29 July 2013; Evaluation: 26 August 2013; Reception of revised manuscript: 15 November 2013; Accepted: 4 December 2013; Available on-line: 6 December 2013
**Portuguese Title and captions by Ulisses M Azeiteiro on behalf of the Journal Editorial Board