Introduction
Endoscopic ultrasound (EUS) has become an important adjunct modality for the diagnosis, staging and therapeutic decision in pancreatic cancer [1, 2]. In large meta-analyses and in comparative studies with other imaging modalities, EUS was found to be the most accurate tool for the detection of small pancreatic masses (≤2 cm), with a pooled sensitivity of over 95% [2, 3].
Tissue acquisition for pathological documentation of pancreatic cancer is mandatory before chemotherapy [4, 5], and increasingly more patients receive such chemotherapy before having a surgical specimen available [6]. Indeed, neoadjuvant therapy is becoming a standard practice for borderline resectable tumours, offering a better chance of achieving a complete surgical resection (R0) [7] and a longer survival after resection [8]. The recent availability of the so-called third-generation fine-needle biopsy (FNB) needles increased significantly the diagnostic yield of EUS-guided tissue acquisition of pancreatic masses [9, 10]. These new FNB needles with cutting edges (crown-tip and fork-tip) are rapidly replacing fine-needle aspiration (FNA) needles in clinical practice given their ability to acquire diagnostic cell blocks in approximately 95% of cases with only two dedicated passes, obviating the need for rapid onsite evaluation [9, 11]. Histological samples can be reliably acquired with these new needles, allowing to perform ancillary testing, such as immunohistochemistry and molecular profiling of the tumour [9-11]. In the era of neoadjuvant chemotherapy and personalised cancer Medicine, EUS-FNB has therefore become a powerful tool in the management of pancreatic cancer [1]. On the one hand, it is an important adjunct to multi-detector computed tomography (CT) and magnetic resonance imaging (MRI) in the multimodality staging of pancreatic cancer and, on the other hand, it allows a safe and effective core tissue acquisition for histology, assessment of tumour markers and tumour gene profiling [1, 9-11].
In this article, the authors present an updated perspective of the place of EUS-FNB in the staging algorithm of pancreatic cancer. Data supporting the increasing role of neoadjuvant therapy for non-metastatic pancreatic cancer and the growing importance of a patient-tailored treatment selection based on tumour subtyping and genetic profiling are also discussed.
A literature search was performed until March 2020, using PubMed Central, Scopus and Google, using the key words and phrases: “pancreatic cancer/adenocarcinoma staging,” “endoscopic ultrasound,” “fine-needle biopsy,” “neoadjuvant chemotherapy,” “personalized cancer Medicine” and “precision therapy.” Prospective/comparative studies, meta-analyses and international consensos guidelines were preferred.
Anatomic Staging, Clinical Staging and Therapeutic Decision by Stage
Pancreatic cancer staging includes:
The classification of the tumour stage, using preferably the 8th edition of the American Joint Committee on Cancer (AJCC) classification (2017) (Table 1) [12]. The primary goal of the revised AJCC criteria is providing information on prognosis, rather than guiding patient management [12].
Table 1. The TNM staging system (7th and 8th editions) from the American Joint Committee on Cancer (AJCC)
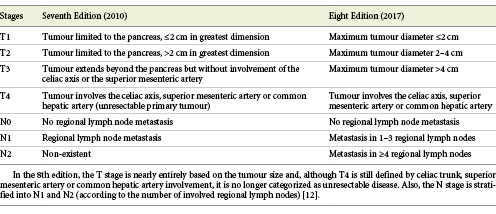
The precise description of the relationship between the tumour and the portal vein/portal venous confluence, superior mesenteric vein, superior mesenteric artery, celiac trunk and common hepatic artery [4]. An imaging staging reporting template should preferably be used to ensure complete assessment of all imaging criteria essential for optimal staging, which will improve the decision-making process [13].
The determination of the clinical stage and the resectability status (based on the integration of the imaging test results and eventual staging laparoscopy), which impacts on therapeutic decision-making, considering 4 stages [4]:
(a) Metastatic disease, which generally benefits from palliative chemotherapy.
(b) Locally advanced disease, also with indication for chemotherapy, due to local vascular spread that precludes complete resection. However, about one-third of these patients may have significant tumour shrinkage with chemotherapy allowing secondary resection of the primary tumour [14]. Hence, in the 8th edition of the AJCC classification, the T4 stage is no longer classified as unresectable [12].
(c) Borderline resectable disease, with indication for neoadjuvant chemotherapy, usually followed by resection surgery.
(d) Resectable disease, usually treated with primary resection surgery, although neoadjuvant chemotherapy with secondary surgery is increasingly being performed in this stage [4,5].
The classification system for resectability based on tumour-vessel relationships (which categorises the tumour in resectable, borderline resectable and unresectable) correlates with the likelihood of achieving a R0 resection and the most widely accepted criteria are from the National Comprehensive Cancer Network (NCCN) [4]. The anatomic definition for borderline resectable cancer, which often requires vascular reconstructions for resection and carries a high risk of positive margins, justifying preoperative chemotherapy, is variable across different classifications systems. The recent guidelines of the American Society of Clinical Oncology (ASCO) categorise as “borderline resectable” any tumour-vascular contact (of any degree), recommending neoadjuvant therapy instead of primary resection [15,16]. It must be highlighted that the anatomic criteria for resectability (determined by imaging findings alone) are clearly insufficient, as a significant proportion of patients with tumours classified as primary resectable ultimately have positive margins and residual disease following resection [17]. Therefore, additional criteria were added in the decision for neoadjuvant chemotherapy (instead of primary surgery) for potentially resectable disease, including biological factors (CA19.9 >500 IU/mL and suspicion of regional lymph node metastases) and clinical criteria (as the presence of persistent “pancreatic pain,” significant weight loss and poor general condition, with ECOG performance status ≥2) [4,17].
The Era of Neoadjuvant Chemotherapy and Personalised Medicine
All imaging modalities tend to understage pancreatic cancer and even patients with R0 resection have poor outcomes, with nearly half of them experiencing tumour recurrence in the first postoperative year [18]. Guidelines endorsed by the European Society for Medical Oncology (ESMO) indicate that only patients with a high probability of complete (R0) resection should receive upfront surgery [19]. The recent expansion of the indications for neoadjuvant chemotherapy is the result not only from a new perspective of “borderline resectable cancer” (integrating clinical criteria and data related to biological behaviour of the tumour) [4,17], but also from emerging data of phase III trials (PREOPANC-1 e Prep-02/JSAP-05), pointing out to a potential benefit of neoadjuvant therapy even in resectable disease [7,20]. The Dutch PREOPANC-1 trial, which included patients with resectable and borderline resectable disease, showed a significant increase in the R0 resection rate, longer disease-free survival and lower rates of metastatic lymph nodes in patients who underwent neoadjuvant therapy when comparing with those receiving upfront surgery (R0 resection rate was 40% after upfront resection versus 71% after neoadjuvant therapy) [7]. Another recent prospective randomised trial of patients with borderline resectable disease was closed prematurely when an interim analysis found a superior median overall survival of 21 months for neoadjuvant therapy versus 12 months for upfront surgery [8]. Neither of the previously mentioned trials [7,8] evaluated the impact of neoadjuvant therapy with FOLFIRINOX, a more effective regimen than gemcitabine alone in the metastatic setting [6]. In three ongoing trials evaluating neoadjuvant FOLFIRINOX in patients with resectable pancreatic cancer [21-23], it is expectable that even better results regarding the overall survival will be achieved in the neoadjuvant FOLFIRINOX group [6].
Neoadjuvant chemotherapy has several advantages: (1) improved local tumour control, increasing the likelihood of negative-margin (R0) resections; (2) identification of patients with rapidly progressive disease, who can then be spared from the morbidity associated with a potentially useless surgery; and (3) optimisation of the access to chemotherapy, taking into account that after a major pancreatic resection surgery, up to 40% of patients are too ill to receive adjuvant chemotherapy [18,24]. Therefore, there is growing evidence favouring preoperative chemotherapy also in potentially resectable disease, with some authors advocating its use in all cases of pancreatic cancer, with the exception of T1N0-stage (stage Ia: tumour limited to the pancreas ≤2cm) [25].
The 2020 NCCN guidelines recommend tumour gene profiling for patients with locally advanced or metastatic disease who are candidates for anti-cancer therapy to identify potentially targetable mutations [4]. The NCCN advocates that testing for actionable somatic findings should be considered prior to chemotherapy in these patients, specifically including, but not limited to: fusions (ALK,NRG1,NTRK,ROS1),mutations (BRAF,BRCA1/2,HER2,KRAS,PALB2), and mismatch repair deficiency [4]. Pathological confirmation of the diagnosis of pancreatic ductal adenocarcinoma is mandatory before chemotherapy, and the NCCN recommends obtaining a core biopsy, if possible, to acquire adequate tissue for ancillary studies (immunohistochemistry and molecular profiling) [4].
Pancreatic cancer diagnosis must be precise and, preferably, should be confirmed preoperatively by EUS-guided tissue sampling, as, in reference centres, nearly 7% of patients undergoing pancreatoduodenectomy for suspected malignancy ultimately have a benign disease [26]. As we step forward to a more frequent use of neoadjuvant chemotherapy and to personalised cancer treatment, the importance of EUS-guided core biopsy also increases.
Whole genome sequencing of pancreatic cancer has shown frequent chromosomal rearrangements leading to gene disruption, affecting genes known to be important in pancreatic cancer development as new candidate drivers of pancreatic carcinogenesis [27]. Over the last decade, multiple study groups have characterised the complex molecular landscape of pancreatic cancer and have described distinct disease subtypes [28]. Five subtypes of pancreatic cancer have recently been defined analysing gene expression features from both tumour and stromal compartments, which were shown to have distinct biology and prognostic implications [29].
The majority of pancreatic cancer patients are still treated with standard (non-target) combination chemotherapy regimens [30]. The difficulty in identifying targets for anti-cancer therapy in pancreatic cancer has been mostly related to three relevant issues: (1) pancreatic cancer has long been thought to be a monomorphic disease, largely defined by 4 non-targetable genomic alterations (in KRAS, TP53, CDKN2A, and SMAD4), with a high heterogeneity of additional mutations, present at low-level frequencies [30,31]; (2) the difficulty in obtaining adequate tumour samples as a source of genomic material for molecular studies [30-32]; (3) the technological demands and prolonged period of time to receive results from molecular studies, precluding a timely result for clinical application [28]. However, emerging data from translational research and recent technological developments in tumour tissue acquisition and in next-generation sequencing technology support the need of “bringing pancreas cancer into the lab” [30]. Aguirre et al. [33] have recently demonstrated a surprisingly high rate of potentially clinically actionable findings in metastatic pancreatic cancer, including a high rate of druggable oncogene lesions in cases harbouring wild-type KRAS genes. In the COMPASS trial, Aung et al. [34] found potentially targetable genomic alterations in 30% of patients with advanced pancreatic cancer and demonstrated that prospective whole genome sequencing and RNA sequencing can be feasible within an adequate time range for clinical application. Very recently, Singhi et al. [31] found in a large cohort of pancreatic cancer patients (both in formalin-fixed biopsies and surgical resection specimens) targetable genomic alterations in 17% of the cases.
The difficulty in obtaining adequate tumour samples for genomic sequencing has also been for many years an important drawback for molecular profiling of pancreatic cancer, having been traditionally performed on resected primary tumours and, more recently, on image-guided percutaneous core biopsies from metastatic disease [31,33,34]. Pancreatic cancer is comprised of a high-density stroma, and the specimens obtained by EUS-FNA are often considered insufficient, contaminated, composed of poor-quality DNA/RNA and suboptimal for genetic analysis [32]. Recent advances in EUS-guided tissue acquisition, with the availability of third-generation FNB needles, significantly increased the diagnostic yield of EUS-guided sampling of pancreatic masses, providing true histological core tissue with a 20-fold higher area when compared to standard FNA needles [35]. This new generation of cutting needles acquire diagnostic cell blocks in approximately 95% of cases with only two dedicated passes, allowing to perform ancillary testing of the tumour [9-11,35]. With EUS-FNB, an important step was taken towards precision therapy in pancreatic cancer, with emerging data showing that EUS-FNB may acquire sufficient tissue for tumour molecular profiling using next-generation sequencing in more than 90% of the tumours [32]. Tumour molecular profiling may offer a method of identifying potentially targetable genomic alterations, but currently it will only benefit a small proportion of patients [29]. Therefore, direct assessment of prediction of chemotherapy response, using tumour models such as organoids or xenograft models, can be an additional way of tailoring therapy for each patient [36]. Lacomb et al. [37] have recently shown that successful organoid creation was feasible in 84% of EUS-FNB samples from pancreatic cancer patients. Recently, Tiriac et al. [36] performed drug sensitivity profiling of pancreatic cancer in organoid models and elucidated novel functional subtypes that predict chemotherapy sensitivity. Identification of molecular and functional subtypes of pancreatic cancer to predict chemotherapy response is urgently needed to improve treatment outcomes in pancreatic cancer. Given the aggressive behaviour of pancreatic cancer, molecular profiling should be done early in the disease course to guide precise treatment decisions [28]. Molecular profiling and subtyping classification of pancreatic cancer will undoubtedly be the future of pancreatic cancer management, but already holds great meaning in the present, being recommended for patients with advanced disease in the 2020 NCCN guidelines [4].
The Place of EUS in the Staging Algorithm
Several international guidelines on the diagnosis and management of pancreatic cancer (NCCN 2020 [4], NICE 2018 [38], ACR 2017 [39], ASCO 2016 and 2017 [15,16] and ESMO 2015 [19]) recommend multi-detector CT, with a dedicated pancreatic protocol (dual-phase, thin-section image acquisition, followed by multiplanar reformation) as the first-line tool for diagnosis and tumour staging. No other imaging study is required in metastatic pancreatic cancer. Multi-detector CT is recommended as the modality of choice for evaluation of tumour-vessel relationships, being particularly accurate for assessing tumour involvement of the local arterial vasculature [4,15,19,38,39]. However, four important limitations of CT for initial staging must be pointed out: (1) the evaluation of isoattenuating tumours (when tumour attenuation is identical to the normal pancreatic parenchyma), corresponding to 5-17% of all pancreatic tumours; (2) the poor overall performance in detecting and characterising local lymph nodes (with sensitivity values below 30% for regional nodal metastases, independent of the morphological criteria being used); (3) inadequate sensitivity for detecting small (≤10 mm) liver metastases (sensitivity of only 69%, significantly lower comparing to MRI); (4) the assessment of peritoneal carcinomatosis, given the frequent small size and possible miliary distribution of peritoneal metastases [15,18].
As a result, in non-metastatic pancreatic cancer after initial CT assessment, both MRI and EUS can potentially add information in the staging workup, which can be relevant in the therapeutic decision process [1,15,24]. In a recent prospective multicentre study, among patients whose pancreatic cancer was deemed resectable based on initial CT findings, MRI with diffusion-weighted sequences detected liver metastases in 10% of these patients, changing patient management and avoiding unnecessary surgeries [40]. Two recent meta-analyses [41,42] assessed the potential benefit of integrating EUS in pancreatic cancer staging, showing that EUS may be particularly useful in some cases: (1) in the assessment of small (<2 cm) and ill-defined/isoattenuating tumours; (2) in the determination of tumoural involvement of the portal vein and portal venous confluence; (3) in the detection of malignant loco-regional lymph nodes (in N-staging); (4) in the detection of even minimal amounts of ascites, which may be an indirect sign of peritoneal carcinomatosis, eventually justifying a diagnostic staging laparoscopy [1,41,42,43]. A 2017 meta-analysis (including 795 patients), which evaluated the potential benefit of EUS for identifying borderline/unresectable disease after initial pancreatic protocol CT, found that EUS results might change patient management from upfront surgery to chemotherapy in 14% of cases [42].
A meta-analysis including 29 studies and 1,330 patients pointed out to a similar performance between EUS and CT scan for evaluating tumour vascular invasion and predicting resectability [41]. However, several studies suggested that EUS may be more sensitive than CT in assessing venous invasion (in particular portal vein/venous confluence involvement), whereas CT scan is considered more sensitive in detecting arterial invasion (of the celiac trunk, superior mesenteric artery and common hepatic artery) [41,44,45]. There are four EUS criteria to predict tumour vascular invasion: (1) loss of the vessel-parenchymal hyperechoic interface (Fig. 1); (2) vessel wall irregularity or luminal narrowing (Fig. 2a); (3) tumour within the vessel lumen (Fig. 2b); (4) peri-pancreatic venous collaterals in an area of a mass that obliterates the normal anatomic location of a vessel [1,43].
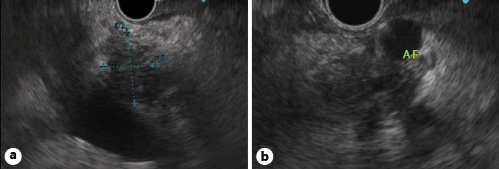
Fig. 1. Linear-array EUS.aHypoechoic mass of the pancreatic head with loss of the hyperechoic interface between the pancreatic parenchyma and the wall of the portal vein.bHypoechoic mass of the pancreatic body with loss of the hyperechoic interface between the parenchyma and the wall of the splenic artery (AE).
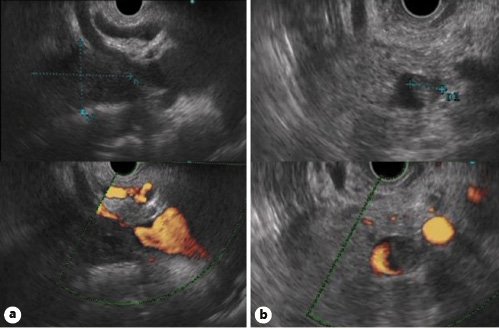
Fig. 2. Linear-array EUS.aHypoechoic mass of the pancreatic head causing luminal narrowing and irregularity of the wall of the portal venous confluence (upper figure: B-mode imaging; lower figure: power Doppler imaging).bIsoechoic mass of the pancreatic body with encasement of the splenic vein and a mass within the vessel lumen (upper figure: B-mode imaging; lower figure: power Doppler imaging).
Pancreatic cancer is characterised by early lymph node spread, and nodal metastases are present in the majority of patients who undergo surgery, even in the absence of morphological criteria for malignant lymphadenopathies assessed by different imaging modalities [39,46]. In a recent meta-analysis evaluating the clinicopathological features of pancreatic cancer according to tumour size (in surgical specimens), lymph node metastases were documented in 79.1% of tumours >2 cm and 64.2% of tumours ≤2 cm [46]. All imaging modalities tend to underestimate nodal metastases, and EUS (with or without FNB) is currently the most accurate modality to determine the N-stage, with a pooled sensitivity of 69% (vs. sensitivity values below 30% for CT and MRI) [1,18,41]. Specificity values for detecting lymph node metastases are similar between EUS, CT and MRI (81-88%) [1, 18,41]. At present, identification of regional lymph nodes suspicious of metastasis (more accurately determined by EUS than by CT or MRI, without necessarily being confirmed by FNB) has a significant clinical impact, as these patients will benefit from neoadjuvant therapy [17].
Response evaluation to neoadjuvant therapy (restaging) is challenging. All imaging studies tend to underestimate the response to chemotherapy, and the diagnostic performance of CT, MRI or EUS is not sufficient to ensure an accurate selection of patients in whom an R0 resection is likely to be achieved [18]. The insufficient performance of imaging studies after chemotherapy is related to the nature of pancreatic cancer, which is composed of extensive and dense fibrous stroma containing varying densities of tumour cells [18]. Chemotherapy may eliminate tumour cells leaving pre-existing fibrotic stroma or may even induce additional fibrosis, which may be mistakenly interpreted on imaging as persistent tumour [18]. PET-CT may add some information in restaging, as a decrease greater than 50% in standardised uptake value (SUV) was shown to be a predictor of R0 resection after neoadjuvant chemotherapy [47]. Taking into account the limitations of structural imaging modalities in restaging, additional studies evaluating the potential utility of functional and quantitative imaging techniques are desirable, such as PET-MRI [48] and CT texture analysis [49].
The potential value of new technologies, such as EUS elastography and contrast-enhanced EUS, as an adjunct to EUS in the diagnostic workup of pancreatic masses remains controversial [1]. The first multicentre study evaluating prospectively the accuracy of EUS elastography for evaluation of pancreatic masses in a large cohort was published 11 years ago [50]. Since then, different studies (all based on strain elastography) reported varied sensitivities and specificities for distinguishing benign from malignant pancreatic masses [51,52]. Strain elastography (both qualitative or quantitative) evaluates the relative elasticity of tissue within a region of interest and lacks objectivity and reproducibility [52]. A recent meta-analysis has found that EUS elastography has a high pooled sensitivity (95%), but a poor pooled specificity (61%) in differentiation of benign and malignant pancreatic masses [51]. A newly developed EUS shear-wave elastography technique will probably improve the accuracy and, most importantly, the reproducibility of EUS elastography in the diagnosis of pancreatic cancer [53]. However, the insufficient specificity of elastography in the diagnosis of pancreatic cancer will probably never be satisfactory due to the fact that stiffness of tissues is not directly related to malignancy, but to various mechanical properties of tissues such as collagen fibre density and distribution [51]. Contrast-enhanced EUS has a higher specificity than strain elastography in the diagnosis of malignancy and may be useful in the characterisation of small (≤2 cm) and ill-defined pancreatic masses, when the enhancement patterns are difficult to depict by CT or MRI [54,55]. The combination of contrast-enhanced EUS and elastography may increase specificity in the differentiation of malignant from benign pancreatic masses, achieving a pooled specificity of 76% [51]. However, the added value of these techniques in the characterisation of indeterminate pancreatic masses after EUS-FNB remains to be proven. In the majority of patients, both EUS elastography and contrast-enhanced EUS add limited value to EUS in the workup algorithm of pancreatic masses, never precluding FNB [1,51,52].
Conclusions
Accurate staging of pancreatic cancer is challenging due to its morphological variability and to the aggressive biological behaviour of this tumour, frequently associated with extra-pancreatic perineural invasion and dissemination to lymph nodes and distant sites, which may be occult or difficult to detect by cross-sectional imaging studies [15,18]. The focus of the initial workup should be to determine the presence of an overt or potentially occult systemic disease on imaging, the likelihood of a complete local resection, and the patient capacity to tolerate available therapies [15]. Each imaging technique has its own diagnostic yield and limitations. A multimodality imaging workup should be pursued as it improves staging accuracy, directing patients with resectable disease to surgery, optimising patient selection to neoadjuvant therapy and avoiding surgical resection in those with more advanced disease [18]. In Figure 3, the authors propose an evidence-based multimodality imaging algorithm for pancreatic cancer staging [4,15,18,19,24,38,39,41,42]. EUS has become a powerful tool in pancreatic cancer management and should be used as an adjunct modality for staging, potentially changing patient management in non-metastatic disease after the initial cross-sectional imaging assessment [1,41,42]. Additionally, it is the method of choice for tumoural sampling [4,15,24], which is playing a pivotal role with neoadjuvant therapy becoming the standard of care in most cases and with the emergence of personalised therapy based on tumour molecular characteristics [4,6,29]. The new generation of FNB needles provides true histological samples in 95% of cases with only two dedicated passes, allowing tumour ancillary testing and molecular profiling [9-11,35].
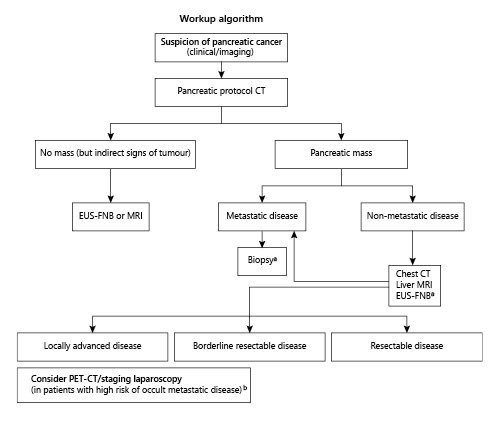
Fig. 3. Multimodality imaging algorithm for pancreatic cancer staging [4,15,18,19,24,38,39,41,42].Key Points: Multi-detector CT, with a dedicated pancreatic protocol, is the first-line tool for diagnosis and tumour staging. No other imaging study is required in metastatic pancreatic cancer, and a biopsy is mandatory before chemotherapy (EUS-guided FNB or image-guided percutaneous biopsy of liver metastases if easily accessible) [4,15,19,24,38,39]. In non-metastatic pancreatic cancer after initial CT assessment, both MRI and EUS can potentially add information to the staging workup (and a chest CT may also be considered to complete staging). MRI may detect liver metastases in 10% of patients whose pancreatic cancer is deemed resectable based on CT findings [40]. The potential incremental benefit of EUS for staging is mostly due to the identification of portal vein/confluence involvement (being particularly valuable in small and ill-defined/isoattenuating tumours), identification of loco-regional nodal involvement and documentation of ascites, eventually justifying a diagnostic staging laparoscopy. EUS also allows FNB for histology and assessment of tumour markers and tumour gene profiling [4,5,9-11,32]. aA core biopsy is recommended to obtain adequate tissue for possible ancillary studies (immunohistochemistry and molecular profiling). Tumour gene profiling is recommended for patients with locally advanced/ metastatic disease who are candidates for anti-cancer therapy [4]. bPET-CT scan and staging laparoscopy may be considered in high-risk patients to detect extra-pancreatic metastases - particularly in borderline resectable and locally advanced disease, but also in potentially resectable disease [4,24]. High-risk features include large primary tumours (>4 cm), suspicious regional lymph nodes, very highly elevated CA 19.9 (>1,000 IU/mL), excessive weight loss and extreme pain [4,24].