Servicios Personalizados
Revista
Articulo
Indicadores
-
Citado por SciELO
-
Accesos
Links relacionados
-
Similares en SciELO
Compartir
Portugaliae Electrochimica Acta
versión impresa ISSN 0872-1904
Port. Electrochim. Acta vol.29 no.3 Coimbra jul. 2011
Miniaturized Membrane Sensors for the Determination of Orphenadrine Citrate
Marianne Nebsen*,1, Mohamed K. Abd El-Rahman1, Amira M. El-Kosasy2, Maissa Y. Salem1, Mohamed G. El-Bardicy1
1Analytical Chemistry Department, Faculty of Pharmacy, Cairo University, Kasr-El Aini street, 11562 Cairo, Egypt
2Pharmaceutical Analytical Chemistry Department, Faculty of Pharmacy, Ain Shams University, Cairo, Egypt
Abstract
Novel miniaturized polyvinyl chloride (PVC) membrane sensors in all-solid state graphite and platinum wire supports were developed, electrochemically evaluated and used for the assay of orphenadrine citrate (ORP). The ORP sensors were based on the formation of an ion-association complex between the drug cation and tetrakis(4- chlorophenyl)borate (TpClPB) anionic exchanger as electroactive material dispersed in a PVC matrix. Linear responses of 10-2 - 10-5 M and 10-2-10-4 M with cationic slopes of 56.4 mV and 53.6 mV over the pH range 4-7 were obtained by using the ORP-coated graphite (sensor 1) and platinum wire (sensor 2) membrane sensors, respectively. The proposed methods displayed useful analytical characteristics for the determination of ORP in Norflex® tablets with average recoveries of 100.01±0.83, 100.09±0.90, and in plasma with average recoveries of 99.4±0.97 and 98.55±0.82, for sensor 1 and 2, respectively. The methods were also used to determine the intact drug in the presence of its degradate and thus could be used as stability indicating methods. The results obtained by the proposed procedures were statistically analyzed and compared with those obtained by using an official method. No significant difference for both accuracy and precision was observed.
Keywords: orphenadrine citrate, potentiometry, stability indicating method, tetrakis(4- chlorophenyl) borate, plasma.
Introduction
Orphenadrine citrate [(RS)-(dimethyl-2-(2-methylbenzhydroxy)ethyl) amine citrate (C18H23NO,C6H8O7, mol. wt. 461.5)] is most widely employed as skeletal muscle relaxant [1]. It acts centrally by depressing the appropriate neurons to prevent the generation of somatic molar nerve impulses. Several methods have been applied in the literature for the determination of ORP in dosage forms and in biological fluids. Techniques such as spectrophotometry [2-6], potentiometry [7], gas chromatography-mass spectrometry (GC-MS) [8-10], atomic absorption [3, 10] and high performance liquid chromatography (HPLC) [11-13], have been used.
From all these procedures only the HPLC technique [13] was recommended as a stability indicating assay. The potentiometric method reported for determination of ORP by ion selective electrode [7], used ammonium reineckate as anionic exchanger and nitrobenzene as plasticizer. This electrode, however, wasn't examined with ORP major degradation product o-methylbenzhydrol, and drugs co-formulated with ORP, such as paracetamol, caffeine and aspirin, and their selectivity coefficients were tested for only a few organic and inorganic cations. Tetrakis(4-chlorophenyl)borate (TpClPB) was reported as famous ion exchanger [14-16]. It was used in the formation of many sensors [17-19]. In this work, it was found that ORP reacted with (TpClPB) to form water insoluble ion association complex. The high lipophilicity and remarkable stability of this complex suggested its selective use as electroactive material in PVC matrix membrane sensors for the determination of the drug studied, in the presence of its degradate and related substances[20].
The aim of this work was to develop simple sensors of low cost with fast response to be applicable in turbid, viscous and coloured solutions. These sensors offered highly sensitive and selective technique for the determination of ORP as a pure drug, in pharmaceutical dosage forms and in the presence of ORP degradation product and co-formulated drugs.
Experimental
Instruments
A Jenway digital ion analyzer model 3330 (Essex, UK) with a Ag/AgCl double junction reference electrode no. 924017-LO-Q11C was used for potential measurements.
Bandelin sonorox magnetic stirrer model Rx 510 S, (Budapest, Hungary) was used during measurements.
A Jenway pH glass electrode No. 924005-BO3-Q11C (Essex, UK) was used for pH adjustments.
Chemicals and reagents
Orphenadrine citrate reference standard was kindly supplied by Egyptian International Pharmaceutical Industrial Company ''EIPICO'', (Cairo, Egypt), its purity was certified to be 99.43 %. o-methylbenzhydrol (degradation product of ORP) was obtained from Sigma chemical company (St. Louis, MO). Its puritywas labelled to be 98.69 %. Norflex® tablets (labelled to contain 100 mg orphenadrine citrate per tablet), were obtained from Egyptian International Pharmaceutical Industrial Company ''EIPICO'', (Cairo, Egypt).
All chemicals and reagents used were of analytical reagent grade. Water used was bi-distilled. Polyvinyl chloride (PVC) high molecular weight, dioctyl phthalate (DOP) and tetrakis (4-chlorophenyl) borate (TpClPB) were purchased from Aldrich (Steinheim, Germany). Tetrahydrofuran (THF) BDH (Poole, England). Hydrochloric acid Prolabo (PA, USA). Britton-Robinson buffer (BRB) (pH 2-12) was prepared by mixing different volumes of 0.04 M acetic acid, 0.04 M phosphoric acid, 0.04 M boric acid and 0.2 M sodium hydroxide [21]. Fresh human plasma was supplied by (VACSERA, Giza, Egypt) and used within 24 hours.
Standard solutions
– ORP stock standard solution (1×10-2 M):
Prepared by transferring 0.461 g of ORP into 100 mL volumetric flask and completing the volume with bi-distilled water using BRB solution to adjust pH at 6.
– ORP working solutions (1×10-6 - 1×10-2 M):
Prepared by suitable dilution from ORP stock standard solution.
– ORP degradate ( o-methylbenzhydrol) standard solution (1×10-3 M):
Prepared by transferring 0.199 g of o-methylbenzhydrol into 100-mL volumetric flask and completing the volume with bi-distilled water using BRB solution to adjust pH at 6.
Procedures
Preparation of electroactive coating membrane: (ORP/ TpClPB /PVC) Five mL 10-2 M aqueous drug solution were acidified with two drops of 1 M hydrochloric acid and mixed with the saturated aqueous solution of TpClPB. The resultant precipitate was filtered, washed with cold water, dried at room temperature (about 20 °C) and grounded to fine powder, forming the ion-pair complex. Elemental analysis was carried out to study the formation of the complex.
In a glass Petri dish (5 cm diameter), 10 mg of the previously prepared ion association complex were mixed thoroughly with 0.35 mL DOP and 0.19 g PVC. The mixture was dissolved in 5 mL THF, and then the Petri dish was covered with a filter paper and left to stand for one hour to allow slow evaporation of the solvent, producing a thick homogeneous master coating PVC solution.
Sensor 1 fabrication (ORP-coated graphite electrode)
A rod of spectrographic graphite (6 mm in diameter and 15 mm long) was inserted in a polyethylene sleeve, and about 3 mm of the other end of the protruded rod served as a measuring surface. This end of the rod was washed with acetone, dried in air for three hours, and dipped rapidly into the previously prepared PVC solution. The solvent was allowed to evaporate in air after each dipping, and the dipping process was repeated 6-8 times to produce a uniform membrane on the surface of the graphite rod. One drop of mercury was added in the polyethylene sleeve to ensure electrical contact with the connection cable. The coated graphite rod was conditioned by soaking in a 10-2 M ORP solution for five hours, and stored in the same solution when not in use.
Sensor 2 fabrication (ORP-coated platinum wire electrode)
The cover of an insulated platinum wire (2 mm in diameter and 10 mm in length) was removed for a length of about 1 cm at both ends. One end of the wire was immersed in the previously prepared PVC solution and was left to stand for 10 min to allow complete air drying, forming a thin membrane around the wire end. The resultant coated wire membrane sensor was conditioned in 10-2 M drug solution for three hours and was stored in the same solution when not in use.
Sensors calibration
The conditioned sensors were calibrated by separately transferring 50 mL aliquots of solutions (1×10-6 - 1×10-2 M) of ORP into a series of 100-mL beakers. The membrane sensors in conjunction with Ag/AgCl reference electrode were immersed in the above test solutions and allowed to equilibrate while stirring. The potential was recorded after stabilizing to ± 1 mV and the emf was plotted as a function of negative logarithm of ORP concentration. The calibration plots obtained were used for subsequent measurements of unknown samples of ORP.
Application to pharmaceutical formulations (Norflex® tablets)
The contents of 10 tablets were weighed, grounded and mixed thoroughly. A suitable portion of powder equivalent to 0.0461 g ORP was transferred into a 100 mL volumetric flask; then 50 mL of bi-distilled water were added. The solution was shaken for 3 minutes before completing to the mark with the same solvent and using BRB solution to adjust pH to 6. This prepared solution is a 10-3 M aqueous solution of ORP. Suitable dilutions were performed to obtain serial of 10-4 to 10-5 M ORP using bi-distilled water and adjusting the pH to 6 by BRB solution. The procedure was completed as described under sensors calibration.
Application to synthetic mixtures containing different amounts of ORP degradate
Aliquots of standard drug solution (10-3 M) were mixed with ORP degradate standard solution (10-3 M) in different ratios. The emf of 50 mL portions of these laboratory prepared mixtures was recorded. Results were computed according to the corresponding regression equation.
Application to plasma
1 mL of 10-2, 10-3 and 10-4 M standard drug solution were added separately into three stoppered shaking tubes (20 mL), each containing 9 mL of plasma which was previously adjusted to pH 6 with BRB solution. The tubes were shaken for 1 minute. The membrane sensors were immersed in conjunction with the reference electrode in these solutions, and then washed with water between measurements. The emf produced for each solution was measured by the proposed electrodes; then the concentration of ORP was determined from the corresponding regression equation.
Results and discussion
Microelectrodes are the subject of much research in recent years and the advantages they offer over conventional electrodes are well known [22,23]. Metallic and graphite-based conductors of many geometric shapes have been suggested, such as wire, disc and cylinders [24, 25]. These electrodes behave as two interface devices, membrane/electrolyte interface and membrane/metal interface [26]. Coated wire electrodes (CWEs) for some cations and anions [28] were described [27-29]. Also coated graphite rods were used as sensors for the determination of some drugs such as atenolol [30], tizanidine [31] and rivastigmine [32].
ORP contains a tertiary amino group and behaves as cation in acidic media – as HCl was added during preparation of electroactive coating membrane – the fact that suggests the use of anionic type of ion exchangers to form complexes with it. TpCIPB with its low solubility product and suitable grain size was found to be optimum for the formation of 1:1 hydrophobic ion association complex with the studied drug, Fig. 1. The complex ratio between TpCIPB and the drug was 1:1 as proved by elemental analysis, Table 1.
Figure 1. Suggested structural formula of ion association complex of ORP with TpCIB.
Table 1. Elemental analysis of ORP-TpClPB complex.
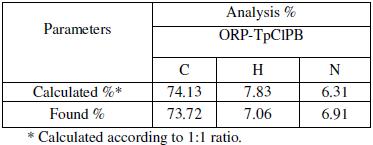
Sensors fabrication
A microsized graphite and platinum wire coated with thin films of PVC-ORPTpClPB were prepared and used as potentiometric sensors for ORP drug. Upon soaking these sensors in 10-1 M drug solution, a homogenous electroactive polymer-ORP site was formed, which induced a potentiometric response for the ORP cation through an ion-exchange mechanism.
It has been reported that PVC acts as a regular support matrix for the membrane and reproducible trap for the ions sensed [33- 35], but its use created a need for a mediator [36]. In the present investigation, DOP (a non polar plasticizer) was found to be the optimum available mediator for the PVC membrane sensors. It plasticized the membrane, dissolved the ion association complexes and adjusted both of the membrane permittivity and ion exchanger sites mobility to give the highest possible selectivity and sensitivity. Except for dibutylsebacate which had the same effect as DOP, other mediators such as, tricresylphosphate and castor oil failed in dissolving the ion association complexes and thus gave noisy responses. The membrane constituents were dissolved in THF that was slowly evaporated at room temperature, leading to the formation of thick homogeneous PVC-ORP-TpClPB solution for coating of both the graphite rod and platinum wire.
Sensors calibration and response time
Electrochemical performance characteristics of the proposed sensors were systematically evaluated according to IUPAC standards [37].
Table 2 shows the results obtained over a period of one month for two different assemblies of each sensor. Typical calibration plots are shown in Fig. 2 and 3. The sensors displayed constant potential readings within ±2 mV from day to day and the calibration slopes did not change by more than ±2 mV per decade over a period of 42 and 18 days for the coated graphite and platinum wire sensors, respectively.
Table 2. Electrochemical response characteristics of the two investigated ORP electrodes.
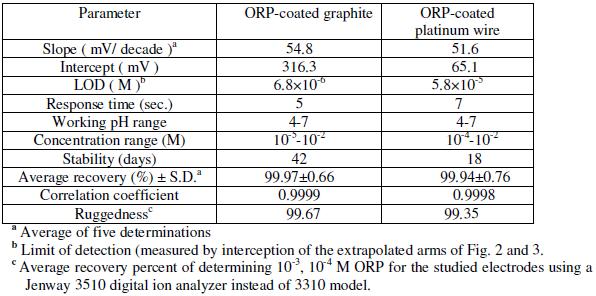
Figure 2. Profile of the potential in mV versus -log concentrations of ORP in M obtained by using sensor 1.
Figure 3. Profile of the potential in mV versus -log concentrations of ORP in M obtained by using sensor 2.
The required time for the sensors to reach values within ±1 mV of the final equilibrium potential after increasing drug concentration 10 folds was found to be 5 and 7 seconds, for sensors 1 and 2, respectively. The slopes of the calibration plot were 54.8 and 51.6 mV/concentration decade, for the coated graphite and platinum wire sensors, respectively. Deviation from the ideal Nernstian slope (60 mV) stems from the fact that electrode responds to the activity of the drug cation rather than its concentration.
Sensors pH and temperature
In measurements with the two investigated sensors, the experimental parameters were studied to reach the optimum conditions. A pH value within the range 4-7 was found to be optimum from the point of view of both sensor function and the chemical form of the test solution, ORP being in the cationic form in acidic media. Fig. 4 and 5 show the potential pH profile for 10-3 and 10-4 M drug solutions. Above pH 8, the potentials displayed by the sensors sharply decrease due to formation of non-protonated ORP. Below pH 4, the potentials displayed by the sensors were noisy and unbalanced due to sensor shocking. It was apparent that the sensors responses are fairly constant in BRB solutions of pH 4- 7, therefore BRB solution of pH 6 was used throughout the measurements.
Figure 4. Effect of pH on the response of sensor 1.
Figure 5. Effect of pH on the response of sensor 2.
Upon studying the effect of temperature, the suggested sensors exhibited slight gradual increase in their potentials as the temperature increased in the range of 20-35 °C. However, the calibration graphs obtained at different temperatures were parallel. The limit of detection, slope and response time did not significantly vary with variation of temperature, indicating reasonable thermal stability of PVC membrane up to 35 °C.
Sensors selectivity
The effect of interfering substances upon the performance of the sensors was studied by separate solution method using the following equation [37]:

where E1 is the potential measured in 10-3 M ORP solution, E2 the potential measured in 10-3 M interferent solution, ZA and ZB are the charges of ORP and interfering ion, respectively, aA is the activity of drug and 2.303RT/ZAF represents the slope of the investigated sensors (mV/concentration decade).
Table 3 shows the potentiometric selectivity coefficients of the proposed sensors in the presence of capsules excipients, degradates, organic and inorganic related substance and also some co-formulated drugs; the results revealed that the proposed membrane sensors displayed high selectivity, and that no significant interference was observed from interfering species. Also, they revealed that sensor 1 displayed greater selectivity for ionic interfering species such as NaCl, KCl, and CaCl2 than did sensor 2.
Table 3. Potentiometric selectivity coefficients (Kpot ORP.I) of the two proposed electrodes by using the separate solutions method (SSM) [37].
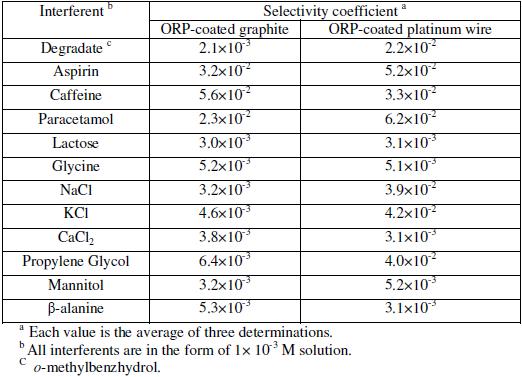
Table 4 shows the results obtained for the determination of ORP in pharmaceutical formulations (Norflex® tablets), proving the applicability of the method, as demonstrated by the accurate and precise percentage recovery; the results obtained were also compared with those obtained by using official method [38] (HPLC method using methanol-0.05 M ammonium phosphate bufferacetonitrile (9:8:3 by volume)). No significant difference in results was found. Placebo experiments containing all additives in the same ratio as that used in tablets were investigated. The excipients present in Norflex® tablets (lactose, glucose, mannitol, sodium chloride, magnesium stearate and polyethylene glycol) did not show any interference. Thus, analysis was carried out without prior treatment or extraction.
Table 4. Determination of ORP in Norflex® tablets by the proposed electrodes and the official method [38].
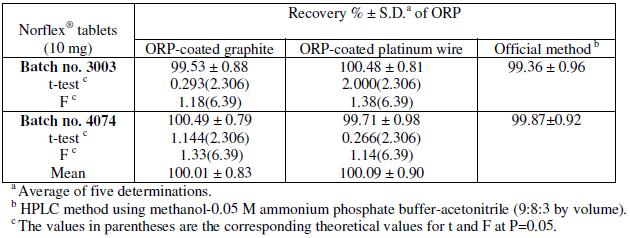
Table 5 shows the results obtained for the determination of ORP in spiked human plasma; it was clear from the results that a wide concentration range of the drug could be determined by the investigated sensors as they gave stable results in slopes and mV readings revealed by the high precision and accuracy of the recovery results. It is also clear from the results shown in Table 5 that sensor 1 is more sensitive than sensor 2 because wider concentration ranges of the drug could be determined.
Table 5. Determination of ORP in spiked human plasma by the proposed sensors.
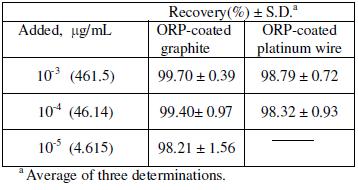
Fig. 6 shows the reported acid degradation of the drug [13]; the degradation products are o-methylbenzhydrol and dimethylethylamine. Table 6 shows the results obtained upon analysis of synthetic mixtures containing different ratios of intact drug to degraded sample, varying from 100:0 to 10:90. The results showed that sensor 1 can be successfully used for selective determination of intact drug in the presence of >90% of the degradate. While sensor 2 suffered from great interference when the degradate concentration is up to about 30 %. Thus sensor 1 is recommended for use in stability-indicating methods.
Figure 6. Reported acid degradation of orphenadrine.
Table 6. Determination of ORP in laboratory prepared mixtures containing different ratios of ORP and its induced acid degradation product by the proposed electrodes.
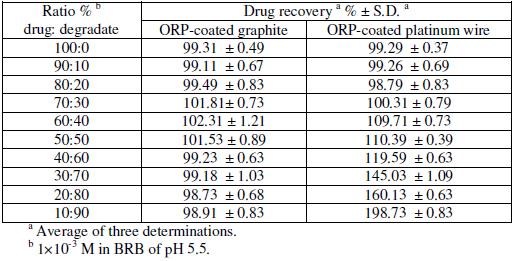
Conclusion
The studied electrodes are sufficiently simple and selective for the quantitative determination of ORP in drug bulk powder, pharmaceutical formulations, plasma and in the presence of its degradate. The use of the proposed sensors offers the advantage of fast response, elimination of drug pre-treatment or separation steps and accuracy over wide concentration range. They can therefore be used for the routine analysis of the drug in quality control laboratories.
References
1. P.P. Gerbino, ''Remington, the science and practice of pharmacy'', 21st Ed., Lippincott Williams & Wikins, Baltimore, Maryland, USA (2005) p.1393. [ Links ]
2. S.A. Shama, J. Pharm. Biomed. Anal. 30 (2002) 1385. [ Links ]
3. H.M. Saleh, M.M. El-Henawee, G.H. Ragab, S.S. El-Hay, Spectroc. Acta Pt. A-Molec. Biomolec. Spectr. 67 (2007) 1284. [ Links ]
4. M.K.S. El-Din, M.A. Abuirjeie, M.H. Abdel-Hay, Anal. Lett. 24 (1991) 2187. [ Links ]
5. R.T. Sane, P.D. Mishra, K.D. Ladage, R.M. Kothurkar, L.S. Joshi, Indian Drugs 26 (1989) 719. [ Links ]
6. A.S. Amin, N.M. El-Kousy, H.A. Dessouki, M. Sultan, Egypt. J. Pharm. Sci. 39 (1998) 463. [ Links ]
7. E.M. Elnemma, F.M. El Zawawy, S.S.M. Hassan, Mikrochim. Acta 110 (1993) 79. [ Links ]
8. A. Pelander, I. Ojanpera, J. Sistonen, I. Rasanen, E. Vuori, J. Anal. Toxicol. 27 (2003) 226. [ Links ]
9. A. Valli, A. Polettini, P. Papa, M. Montagna, Ther. Drug Monit. 23 (2001) 287. [ Links ]
10. A.M. Au, R. Ko, F.O. Boo, R. Hsu, G.Z. Perez, Yang, Bull. Environ. Contam. Toxicol. 65 (2000) 112. [ Links ]
11. H.N. Al-Kaysi, M.A.S. Salem, Anal. Lett. 20 (1987) 1451. [ Links ]
12. S.M. Selkirk, J.H.McB. Miller, G. Smith, A.F. Fell, J. Pharm. Pharmacol. 35 (1983) 23. [ Links ]
13. S.M. Selkirk, A.F. Fell, G. Smith, J.H.M. Miller, J. Chromatogr. 288 (1984) 431. [ Links ]
14. P. Kissinger, Laboratory Techniques in Electroanalytical Chemistry, Marcel Dekker, New York, 1991 (Chapter 4). [ Links ]
15. D. Diamond, Principles of chemical and biological sensors, in: Chemical Analysis, Vol. 150, Wiley, 1998, Chapter 2, p.19. [ Links ]
16. J.L.F.C. Lima, M.C.B.S.M. Montenegro, Mikrochim. Acta 131 (1999) 187. [ Links ]
17. S.S.M. Hassan, R.M. Abdel-Aziz, A.B Abbas, Anal. Chim. Acta 321 (1996) 47. [ Links ]
18. S.S. Hassan, M.M. Amer, S.A. Abdel-Fattah, A.M. El-Kosasy, Talanta 46 (1998) 1395. [ Links ]
19. A.M. El-Kosasy, M.A. Shehata, N.Y. Hassan, A.S. Fayed, B.A. El-Zeany, Talanta 66 (2005) 746. [ Links ]
20. A.M. El-Kosasy, J. AOAC Int. 86 (2003) 15. [ Links ]
21. H.T.K. Britton, R.A. Robinson, J. Chem. Soc. (1931) 1456. [ Links ]
22. M. Shamsipur, F. Mizani, M.F. Mousavi, H. Eshghi, H. Karami, Anal. Chim. Acta 589 (2007) 22. [ Links ]
23. M. Koudelka-Hep, P.D. Van der Wal, Electrochimica Acta 45 (2000) 2437. [ Links ]
24. X. Ji, Baokang Jin, J. Ren, J. Jin, T. Nakamura, J. Electroanal. Chem. 579 (2005) 25.
25. W. Peng, E. Wang, Anal. Chim. Acta 281 (1993) 663. [ Links ]
26. H. James, G. Carmack, H. Freiser, Anal. Chem. 44 (1972) 856. [ Links ]
27. H. Ibrahim, Y.M. Issa, H.M. Abu-Shawish, J. Pharm. Biomed. Anal. 44 (2007) 8. [ Links ]
28. A. Abbaspour, A. Izadyar, H. Sharghi; Anal. Chim. Acta 525 (2004) 91. [ Links ]
29. J.E. Lockridge, N.E. Fortier, G. Schmuckler, J.S. Fritz, Anal. Chim. Acta 192 (1987) 41. [ Links ]
30. P. Cervini, L.A. Ramos, Ã.G. Cavalheiro, Talanta 72 (2007) 206.
31. A.A. Bouklouze, A. El Jammal, J.C. Vire, G. J. Patriarche, Anal. Chim. Acta 257 (1992) 41-48. [ Links ]
32. A.M. EL-Kosasy, M.Y. Salem, M.G. EL-Bardicy, M.K. Abdelrahman, Chem. Pharm. Bull. 56 (2008) 753. [ Links ]
33. J.O'M. Bockris, ''Comprehensive Treatise of Electrochemistry'', Plenum Press, New York (1981), Section 3. [ Links ]
34. N.A. El-Ragehy, A.M. El-Kosasy, S.S. Abbas, S.Z. El-Khateeb, Anal. Chim. Acta 418 (2000) 93. [ Links ]
35. A. Graggs, R. Kataky, D. Parker, Analyst 119 (1994) 181. [ Links ]
36. J. Zyka, ''Instrumentation in Analytical Chemistry'', Ellis Horwood, Chichester, UK, vol.2 1994. [ Links ]
37. IUPAC, Analytical Chemistry Division, Commission on Analytical Nomenclature, Pure Appl. Chem. 72 (2000) 1851-2082. [ Links ]
38. The U.S. Pharmacopoeia, 27th edition, U.S. Pharmacopeial Convention, Rockville, MD, 2004. [ Links ]
*Corresponding author. E-mail address: mariannenebsen@hotmail.com
Received 24 November 2010; accepted 10 June 2011