Serviços Personalizados
Journal
Artigo
Indicadores
-
Citado por SciELO
-
Acessos
Links relacionados
-
Similares em SciELO
Compartilhar
Portugaliae Electrochimica Acta
versão impressa ISSN 0872-1904
Port. Electrochim. Acta vol.35 no.2 Coimbra mar. 2017
https://doi.org/10.4152/pea.201702081
Effect of Operating Parameters on Electrochemical Discoloration of Acid Blue 1 on BDD Electrode
Z.A. Ayoub and M.M. El Jamal*
Inorganic and Organometallic Coordination Chemistry Laboratory (LCIO), Faculty of Sciences (I), Lebanese University, El Hadath, Lebanon
Abstract
The degradation of the AB1 dye by electro-generated species using a BDD electrode was performed. The results were explained by the generation of OH* radical, S2O82- in the presence of sulfate, and active halide species in the presence of halide salt. The discoloration rate increases in this order: sulfate, KCl, KBr. In the presence of KCl, the discoloration is affected by the current density, initial pH, temperature, and concentration of the supporting electrolyte; however, the concentration of the dye and the ionic strength showed a negligible effect. The intermediates produced during the discoloration are a function of the pH of the solution. In the presence of sulfate, the discoloration rate is very slow, and the mechanism of discoloration is different from that in the presence of KCl. The thermodynamic parameters of the discoloration are calculated.
Keywords: BDD electrode; Acid Blue 1; discoloration; kinetic; strong electrolyte.
Introduction
Synthetic dyes are extensively used in various branches of industry: textile, leather tanning, paper production, cosmetic products, and food technology. Different chemical classes of dyes are frequently employed on industrial scale, such as azo, anthraquinone, sulfur, indigo, and triphenylmethyl derivatives. Due to large-scale production and extensive application, synthetic dyes can cause considerable environmental pollution, and are serious health-risk factors [1-4]. For this reason, there is a need to apply powerful methods to ensure the complete discoloration and degradation of dyestuffs and their metabolites present in the spent dyeing baths.
Many studies have been carried out to investigate the degradation of the triphenylmethane dyes, such as adsorption [5, 6], biodegradation [7, 8], photocatalytic degradation [9-12], oxidation with persulfate - E133 [13], crystal violet [14], and E131V [15]-, and finally, electrochemical treatment: alphazurine on BDD electrode [16], crystal violet on BDD [17], methyl violet [18] and malachite green [19, 20] have been investigated.
Advanced oxidation processes involve in situ generation of powerful chemical oxidants, such as OH* and SO4-* for the removal of a wide range of organic contaminants in wastewater. The generation of these oxidants at the BDD electrode surface from the oxidation of an aqueous solution occurred as follows [21-23].

The generation of SO4, and S2O8 in an aqueous solution of sulfate could be represented as follows:

Less powerful oxidants, such as Cl2 and Br2, can also be produced in a solution containing halide salt (X-: Cl-, Br-):

The HOX/OX- pair reacts with organic compounds by addition, substitution or oxidation.
Acid blue 1, also called sulfan blue 5 or E131 VF, is a dark greenish synthetic triphenylmethane dye (Fig. 1).
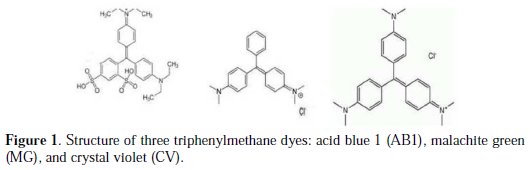
It is structurally very similar to the patent blue V and brilliant blue (three phenyl rings with different substituted groups). The mineralization of the mentioned dyes by persulfate failed [13, 15].
The aim of this work is to study the electrochemical oxidation of aqueous solutions containing acid blue 1 (AB1) using a boron-doped diamond electrode. Thus, it is of interest to study the effect of some experimental parameters, such as the nature of the strong electrolyte, pH, temperature, current intensity, and others, on the indirect oxidation of the acid blue 1 on a BDD electrode.
Experimental
All chemical reagents used were of analytical grade. The food colorant AB1 is used as purchased from Sigma Aldrich (C27H31N2O6S2-Na, purity: 50 %, MW: 565.67 g). Stock solution of acid blue 1 (AB1) was prepared by dissolving 40 mg in one liter of distilled water. The concentration of the dye in the reactional mixture was selected in such a way that the absorbance of the dye followed Beer's law. Most of the electrolysis experiments were done at room temperature (293 K), 2 mA for KBr, 5 mA for KCl and 20 mA for Na2SO4 and pH ∼5, in presence of 8 mg L-1 of AB1, 0.1 M, of the strong electrolyte. The experiments were carried out in a single electrolytic cell.
BDD electrodes are bipolar plates (50×25×2 mm) from NeoCoaT (Switzerland). The distance between the two electrodes was 5 cm (undivided cell). The electrolysis was done with a Chrono-Amperostat, type CEAMD-6, from Taccusel. Measurements of pH were carried out using a Schott Gerate CG 819 pH-meter.
The discoloration rate of the food colorant was followed by measuring the absorbance at the maximum wavelength of AB1 (640 nm). UV-visible spectra were recorded on a double beam UV-visible spectrophotometer, in order to detect any shift in the λmax or the appearance of a new band.
Results and discussion
Nature of the supporting electrolyte
The strong electrolyte added to do the electrolysis has a great importance, since, in the first step, it is oxidized at the anode, and in the second step it probably oxidizes the organic compounds present in the solution. Therefore, the intermediates and the final products are a function of the strong electrolyte present in the medium. Four strong electrolytes are used in this study: KCl, KBr, KI and Na2SO4. The electrolysis in the presence of KI produces I2, which is a weak oxidant and, therefore, is not able to attack AB1 dye [the absorbance at 640 nm (λmax) did not vary with the electrolysis].
Electrolysis in the presence of KCl
Order with respect to AB1
In the presence of KCl, the absorbance at 640 nm decreases with the time of electrolysis (Fig. 2), but at 480 nm increases.
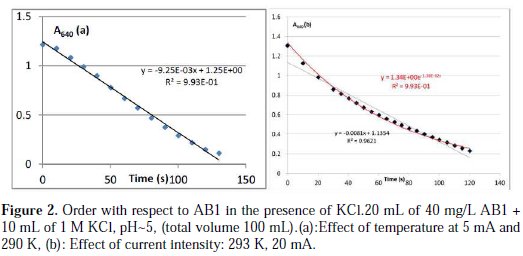
The time required for total discoloration or degradation is a function of the operational parameters which will be later discussed. A detailed discussion about the evolution of the UV- visible spectrum is given in the paragraph on the effect of pH. At the pH (pH∼5) of the dye solution, the reaction occurred into two steps: the first one is fast and corresponds to a color change of the solution from deep blue to slight pink; and in a second step total discoloration occurred (slow step). So, the rate constant calculated in these conditions corresponds to the rate constant of the first step. The order with respect to AB1 is not clear; in fact, there is a strong competition between order zero and one. When studying the effect of temperature and the ionic force, the best order is zero (Fig. 2a), but when studying the effect of KCl concentration and current intensity, the best order is 1 (Fig. 2b), and finally, when studying the effect of dye concentration, for a given concentration, the best order is zero, and for other the best order is 1.
Effect of the food colorant concentration
At BDD electrode, the discoloration rate constant, ko,obs (slope of the line A640 vs. time), is approximately the same when the concentration varies in the range from 2 to 8 mg L-1 (4 different mixtures were prepared for this study); so, the rate constant ko,obs (ki for order i) does not vary with AB1 concentration, but the time needed to have total discoloration is proportional to the concentration of AB1.
Effect of pH
The UV-visible spectrum of AB1 aqueous solution shows three bands at 312 nm (smallest one), 414 nm, and 640 nm (highest one). AB1 has an acid-base property with pka equal to 2.2. In pH lower than 1, the AB1 solution is yellow (λmax: 414 nm), and it is blue in pH higher than 3 (λmax: 640 nm).
In 0.1 M H2SO4: The solution is yellow. There is only one important band at 416 nm and a small one at 274 nm. During electrolysis, there is a decrease in A414 accompanied by a progressive blue shift to 346 nm, whereas the absorbance at 274 nm remains ∼ constant. The yellow color of the solution faded slowly. According to the literature, the progressive blue shift is attributed to the dealkylation of the amino groups and partial degradation of the dye (Fig. 3a) [24].
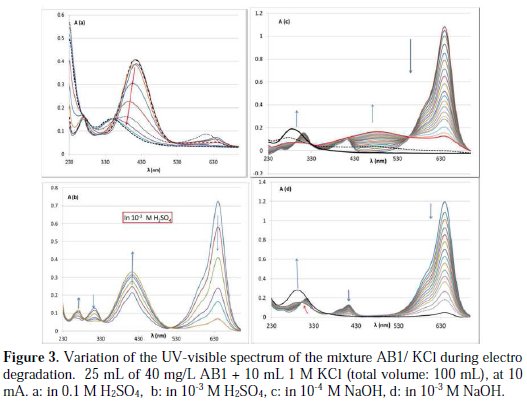
In 0.01 M H2SO4: At the beginning, the visible spectrum shows two bands at 414 (more intense) and 640 nm. In the first step of electrolysis, the absorbance at 640 nm decreases, but at 414 nm increases; then, the absorbance at 640 nm begins to increase with a blue shift to 628 nm, and at 414 nm continues to increase. At the end, the bands at 414 and 628 nm begin to decrease with a progressive blue shift to 604 nm (spectra not shown here).
In 10-3 M H2SO4: At first, the solution changes with time from green to yellow, and then it becomes slowly transparent. The A640 and A314 decrease, whereas A414 and A272 increase to reach a maximum (Fig. 3b). The increase in A414 (λmax of the acidic form) isn't because of the decrease in the pH of the solution, since there is a reduction of H+ on the cathode. Then, the absorbance at 640 begins to increase with a progressive blue shift to 604 nm, and at 414 begins to decrease. There are several isobestic points at 284, 328 and 510 nm. At the end, the absorbance at 640 and 414 nm decreases, but the absorbance below 370 increases with the appearance of a new band at 330 nm (the spectra of the second step are removed for clearness of the figure).
In water and in less basic medium (10-4 M NaOH): The evolution of the UV- visible spectrum in both mediums is approximately the same - the spectrum shows three bands at 314, 414, and 640 nm. A new large band appears with time (350 nm - 558 nm). At first, the solution changes from deep blue to slight pink, and then it becomes slowly transparent. There is a decrease in A640 and A314 nm, but an increase in A480 and A274 nm; the absorbance at 414 nm remains ∼ constant with time (Fig. 3c). The decrease in A640 nm is linear with time, so, the order with respect to the discoloration of AB1 is zero. The rate constant decreases with the increase in pH. There are also three isobestic points at 294, 332 and 558 nm. After a certain time, the absorbance at 480 nm begins to decrease, and the absorbance at 640 nm continues to decrease, accompanied by the appearance of a new band at 274 nm (Fig. 3c).
In basic medium (10-3 M NaOH): The UV-visible spectrum shows three bands at 314, 414, and 640 nm. These three bands decrease during electrolysis (Fig. 3d). The color of the solution passes from deep blue to transparent, without passing through the pink color. The same trend is observed in the presence of sulfate at the pH of the dye solution. Comparing the evolution of the UV-visible spectrum of AB1during electrolysis with that in ref. 20, we can say that total degradation of AB1occurred in basic medium in the presence of KCl.
It is obvious that the pH of the medium is an important parameter which affects the mechanism of the reaction. HPLC MS will be helpful to determine the mechanism of the indirect oxidation of AB1.
The comparison between the AB1 dye, crystal violet (CV) and malachite green (MG), with respect to the evolution of the UV-visible spectrum, shows different behaviors: in the case of CV, the solution changes from pink to faded blue during electrolysis.
The decrease in A590 is accompanied by a bathochromic shift, due to the substitution of chlorine on the phenyl ring(s), or the deamination of one amino group (leading to an increase in resonance as in MG), then, degradation of the dye (Fig. 4a)).
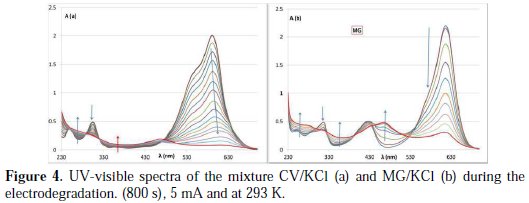
In the case of MG, which has two amino groups, the behavior is similar to AB1; in the first step, there is an increase in the region between 430 and 500 nm, and a decrease in the band at 640 nm, and the absorbance at 426 remains ∼ constant (Fig. 4b).
Effect of the current intensity
Current intensity is also an important parameter in electrolysis. The generation of chlorine species by electrolysis was done at pH (pH∼5)of the dye solution in the presence of 8 mg L-1 of AB1 and 0.1 M KCl (as final concentrations), at five constant currents ranging from 1 mA to 20 mA. As expected, the degradation rate constant increases linearly with the increase of the current intensity (k1obs×10>3 = 0.688 × I(mA), R2: 0.997). The increase in the degradation rate is related to the increase in the production rate of chlorine species. Similar results were obtained with other organic compounds [25, 26].
Effect of KCl concentration
The effect of KCl concentration on the degradation rate was undertaken in the following conditions: 0 M ≤ [KCl] ≤ 0.2 M (KCl added), 8 mg L-1 of AB1, pHo∼ 5, 293 K, with 5 mA. The rate constant increases linearly with the increase in KCl concentration (k1,obs x103: 0.87x [KCl](M)+ 2.5, R2: 0.986)(k1,obs: slope of the line lnA vs. time). The reason is that a higher amount of chlorine/hypochlorite will be generated, while increasing the chloride concentration, due to the increased mass transport of chloride ions to the anode surface [25]. This result confirms the discoloration and the degradation of the organic compounds via the electro-generated chloride species [26, 27]. The discoloration of the rate constant is not negligible when the volume of KCl added is zero, since the powder of AB1 contains already sulfate and chloride as impurities.
Effect of the ionic force
In the presence of a constant concentration of KCl (0.1 M), the rate constant Ko remains approximately constant with the increase of the ionic force by addition of several volumes of 1 M Na2SO4 (7 different mixtures were prepared for this study). Maybe this is because the powder of the food colorant already contains 50 % of inorganic salts (sulfate and chloride).
Effect of temperature
In general, any increase in temperature decreases the solubility of Cl2 in water and, therefore, decreases the discoloration rate constant of the dyes [28]. The effect of the temperature on the degradation rate was investigated with the conditions mentioned in the caption of Fig. 5a.
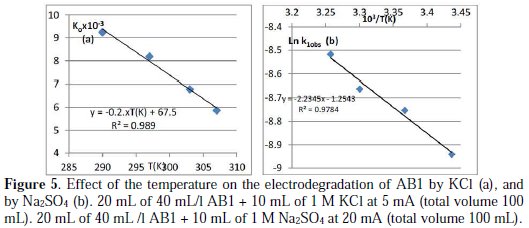
For the range of temperature between 280 and 303 K, ko decreases linearly with the increase of temperature (Ko × 103= -0.2×T(K) + 67.5, R2:0.989).
In the presence of KBr
In the presence of KBr, the decrease in the A640 is four times faster than in the presence of KCl.
At pH (pH∼ 5) of the dye solution, the general shape of the spectrum vs. time is similar to that in the presence of KCl: there is a decrease in the absorbance at 640 nm (λmax), accompanied with an important increase in the absorbance in the zone from 414 to 560 nm (new band, λmax: 480 nm) (Fig. 6a).
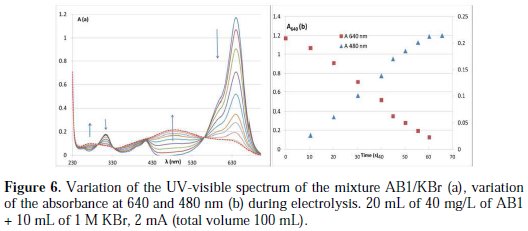
The discoloration passes by two steps: the first one is fast and corresponds to a change of color from blue to brown pink; the second one is slower and corresponds to total discoloration. In the first step, the absorbance at 480 nm increases linearly with time. By varying the concentration of KBr in the medium, the order of the first step with respect to AB1 remains zero (Fig. 6b), and the rate constant remains ∼ constant (five different mixtures were prepared for this study). In the absence of KBr, the rate constant is not zero, since the sample already contains a strong electrolyte as impurities (50 %).
The discoloration in the presence of sulfate is very low with 5 mA; for this reason, the discoloration is done with 20 mA (more than 2 h are needed to have total discoloration, with 20 mA, Fig. 7).
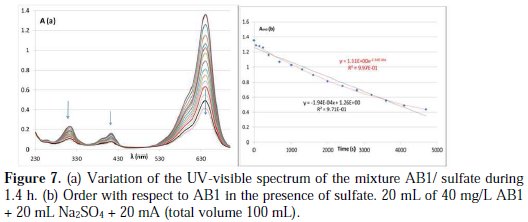
In the same conditions, the rate constant is 40 times lower than in the presence of KCl. The behavior in the presence of sulfate is completely different, with no new band or isobestic point observed, but with a decrease in the whole spectrum with time (Fig. 7a). The blue color faded progressively; no intermediate color is observed during the reaction, as was the case with KCl and KBr, where the blue color of AB1 turns pink to brown with time, and then becomes transparent. The best order with respect to AB1 is order 1 (Fig. 7b) (similar order is observed with malachite green [20]). According to the literature, in the presence of sulfate, AB1 undergoes total discoloration [17]. With a constant concentration of sulfate (0.05 M), the increase in the food color concentration leads to a negligible decrease in the k1obs rate constant. The increase in the concentration of sulfate in the medium from 0.05 to 0.4 M has no effect on the K1obs rate constant. The increase in pH from 1 to 4 causes a slight increase in the rate constant, from 1.35×10-4 to 2.08×10-4 (four different mixtures were prepared to study every parameter). Similar results are obtained with the electrodegradation of CV on the BDD electrode [17]. Finally, the increase in temperature increases the degradation rate of AB1 (Fig. 5b). Similar effect is observed with other organic compounds on BDD electrode [29, 30].
The activation parameters associated with the discoloration are calculated as follows: the plot of ln k1obs vs. 1/T gives the value of the activation energy (Ea), according to Arrhenius equation:

The ΔH#, ΔS# and ΔG# values can be calculated from the two equations:

The activation energy and the other kinetic parameters in the range of temperature studied (18 °C - 34 °C) are listed in Table 1.
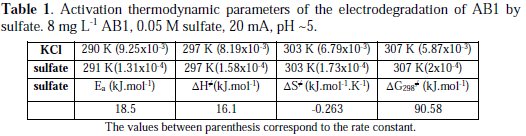
Conclusions
At BDD electrode, the discoloration of AB1 in the presence of sulfate is too much slower than in the presence of KCl and KBr. The increase in the current intensity, the dye concentration, and the ionic force follow identical behaviors in both kind of salts, but the same does not happen with respect to the temperature. Total degradation occurred in the presence of sulfate and in the presence of KCl in a basic medium. In the presence of KCl, the pH of the medium strongly affects the reaction mechanism; HPLC MS will help us have an idea about the intermediates of the reaction at different pH and confirm if there is total degradation.
References
1. Robinson T, McMullan G, Marchant R, et al. Bioresource Technol. 2001;77:247. [ Links ]
2. Fonovich T M. Drug Chem Toxicol. 2012;36:343. [ Links ]
3. Inetianbor J E, Yakubu J M, Ezeonn SC. Asian J Sci Technol. 2015;6:1118. [ Links ]
4. EFSA Journal, Parma, Italy, 2013:11(3):2818, ''Re-evaluation of Patent Blue V (E 131) as a food additive''
5. Rammal R S, Zatiti S A, El Jamal MM. JUCTM (Sofia). 2011;46:283. [ Links ]
6. Bulut E, Ozacar M, Sengil I A. Microp Mesop Mater. 2008;115:234. [ Links ]
7. Lucova M, Hojerova J, Pazourekova S, et al. Food Chem Toxic. 2013;52:19. [ Links ]
8. Chaube P, Indurkar H, Moghe S. Asiatic J Biotech Res. 2010;1:45. [ Links ]
9. Diao Z-H, Xu X-R et al. Sep Purif Technol. 2015;154:168. [ Links ]
10. Barka N, Qourzal S, Assabbane A, et al. Chem Eng Comm. 2011;198:1233. [ Links ]
11. Chen C-C, Fan H-J, Jan J-L. J Phys Chem C. 2008;112:11962. [ Links ]
12. Saquib M, Tariq M A, Faisal M, et al. Desalination. 2008;219:301. [ Links ]
13. Gosetti F, Gennaro M C, et al. J Chromatography A. 2004;1054:379. [ Links ]
14. Fayoumi L M A, Ezzedine M A, Akel H H, et al. Port Electrochim Acta. 2012;30:121. [ Links ]
15. Elddine H A N, Damaj Z K, Yazbeck O A, et al. Port Electrochim Acta. 2015;33:275. [ Links ]
16. Nava J L, Quiroz M A, Martinez-Huitle C A. J Mex Chem Soc. 2008;52:249. [ Links ]
17. Palma-Goyes RE, Torres-Palma RA, et al. Chemosphere. 2010;81:26. [ Links ]
18. Hamza M, Abdelhedi R, Brillas E, et al. J Electroanal Chem. 2009;627:41. [ Links ]
19. Rao A N S, Venkatarangaiah V T. Port Electrochim Acta. 2014;32:213. [ Links ]
20. Guenfoud F, Mokhtari M, Akrout H. Diamond Related Mater. 2014;46:8. [ Links ]
21. Martinez-Huitle C A, Alfaro M A Q, J Environ Eng Manag. 2008;18:155. [ Links ]
22. Enache T A, Chiorcea-Paquim A-M, Fatibello-Filho O, et al. Electrochem Comm. 2009;11:1342. [ Links ]
23. Souza R B A, Ruotolo LAM. Int J Electrochem Sci. 2013;8:643. [ Links ]
24. Chen C-C, Lu C-S, Mai F-D, et al. J Haz Mat. 2006; B137:1600. [ Links ]
25. Rajkumar D, Jong G K. J Haz Mat. 2006;B136:203. [ Links ]
26. El Jamal M M, Mousaoui A M, Naoufal D M, et al. Port Electrochim Acta. 2014;32:233. [ Links ]
27. Tabarra MA, Mallah HA, El Jamal MM. J Chem Technol Metall (Sofia). 2014;49:12. [ Links ]
28. Schmittinger P. Chlorine. In: Ullmann's Encyclopaedia, vol. 6A. Weinheim: VCH; 1986. P.399. [ Links ]
29. Chen T-S, Kuo Y-M, Chen J-L, et al. Int J Electrochem Sci. 2013;8:7625. [ Links ]
30. Chen T-S, Huang K-L. Int J Electrochem Sci. 2013;8:6343. [ Links ]
Acknowledgements
The author thanks the Lebanese University for providing financial assistance to carry out this work.
*Corresponding author. E-mail address: mjamal@ul.edu.lb
Received June 30, 2016; accepted December 9, 2016