Introduction
Mild steel is a widely used construction material in numerous different industries. This is mainly due to its exceptional mechanical properties and minor costs, compared to other materials [1]. However, mild steel, due to its oxidation potential, is predisposed to corrosion in an aqueous medium; therefore, its surface must be protected [2]. Iron and mild steel protection can be achieved either by using classic inhibitors [1,3,6] or chemical or electrochemical surface modification methods, such as SAM's (Self-Assembled Monolayers) composed of silanes [7], phosphonic acids [8] or electrochemical reduced aryldiazonium salts [9,10-12 ]. In general, most inhibitors are organic molecules containing heteroatoms such as nitrogen, oxygen, phosphorous, sulfur, etc. It has been well established that these molecules performance towards the corrosion protection decreases following the order: O > N > S > P [4, 6].
As (7-Amino-8-methylphenoxazin-3-ylidene)-diethylazanium dichlorozinc dichloride chemical structure possesses several adsorption sites (a phenoxazin ring rich in electrons, O, N atoms, etc.), it is an interesting molecule for testing its corrosion inhibition properties towards mild steel [13]. Density functional theory has been developed to a convenient method, to decrypt experimental results and to associate them with the structural parameters of the studied molecular systems, thereby giving an ideal opportunity to understand the corrosion process at the molecular level [14-15 ]. This method made it possible to correctly predict the inhibition efficiency of organic corrosion inhibitors, based on their electronic and molecular properties. In this study, Brilliant Cresyl Blue (BCB) was used as corrosion inhibitor for mild steel in a hydrochloric acid solution (c= 0.5 M). The adsorption mechanism was studied using Monte Carlo calculations [16-17 ].
Experimental
For the electrochemical measurements, the electrode was prepared by embedding a mild steel wire (d = 1 mm, l = 15 mm) inside a Teflon®(d = 1 cm, l = 8 cm) tube using an epoxy resin. Before the use, the electrode surface was polished using silicon carbide abrasive paper; then, it was placed on a (DP-Nap) cloth with an aluminum oxide (0.3 micron particle size) suspension, washed and sonicated in water. The chemical composition of the electrode was as follows: Fe 99.54%, C 0.12%, P 0.03%, Mn 0.18%, silicon 0.05%, Cr 0.01%, S 0.02%, Mo 0.01% and Ni 0.001%.
Electrochemical measurements
A PalmSens potentiostat was used in combination with a three-electrode cell, at 298K. A graphite rod (d = 5 mm, l = 8 cm) served as an auxiliary electrode, and the saturated calomel electrode (SCE) was the reference electrode. The potentiodynamic polarization curves were obtained starting from an electrode potential, cathodically and anodically, of at least 250 mV vs EOCP, with a sweep rate of 1 mVs−1. The measurements were conducted under atmospheric conditions.
Monte Carlo calculations
The molecular model for the BCB was built using Avogadro software [18]. Materials studio 7.0 was used to construct a Fe(1 1 0) slab model.
The iron surface with (1 1 0) orientation was based on Bravais-Friedel-Donnay-Harker (BFDH) crystal morphologies calculation (Fig. 1A), as the most stable iron surface [19].
The MC calculations of the interaction between BCB and the iron surface were carried out in the simulation box of Fe((1 1 0) - 29.789Å × 29.789Å × 6.409 Å (Fig. 1)), under periodic boundary conditions (PCB), with a 45Å vacuum layer along the C-axis.
The corrosion medium effect on MC calculations was replicated by loading 150 water molecules, 20 hydronium ions and 20 chloride ions (geometrically optimized using Universal forcefield) onto the simulation box, together with one or two BCB molecules (using the same optimization algorithm). Before calculations, the charges for all optimized molecules were assigned using the Gasteiger method [20] (Fig. 2).
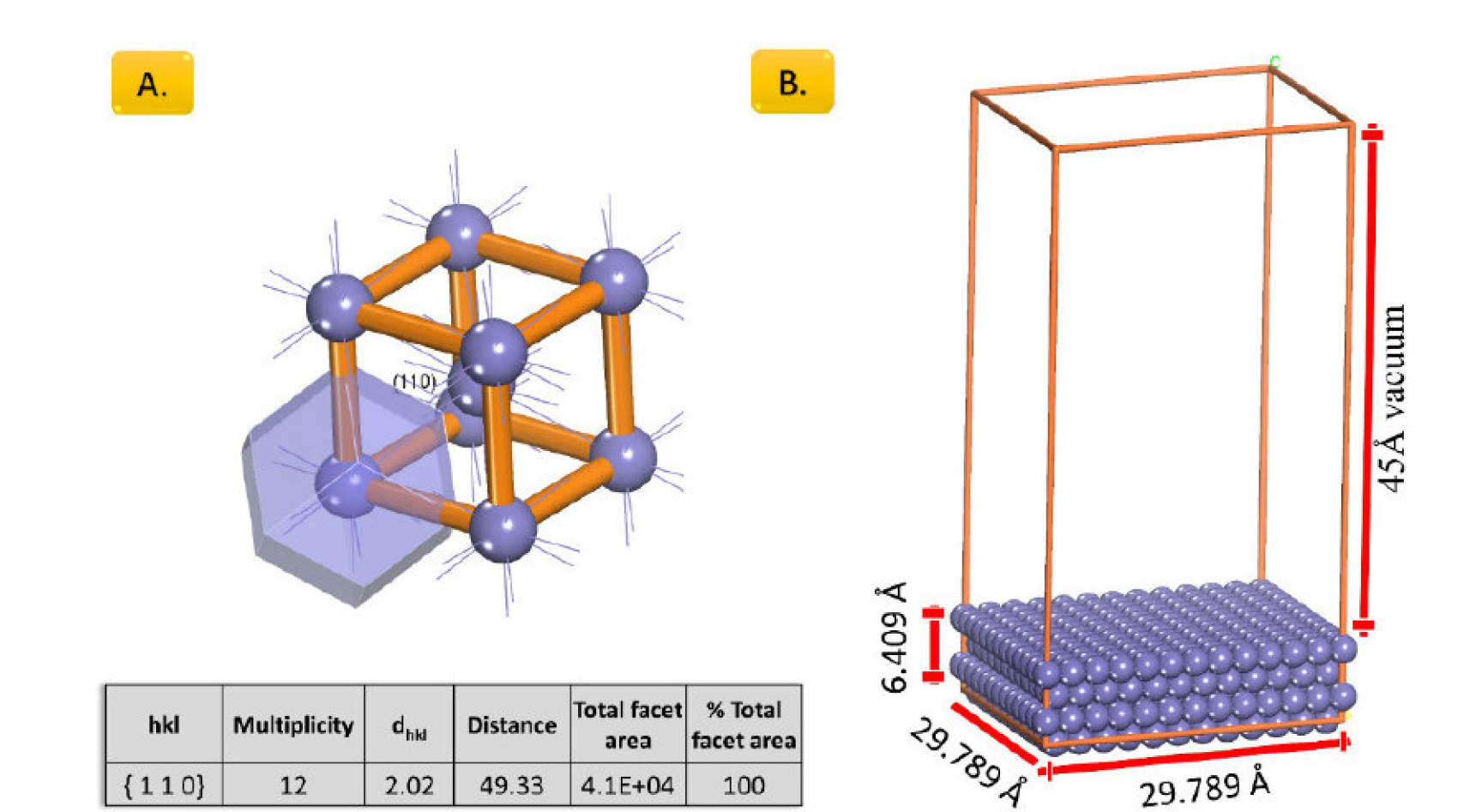
Figure 1 A. BFDH results for iron unit cell and B. Dimensions of the Fe (1 1 0) slab model used for MC calculations.
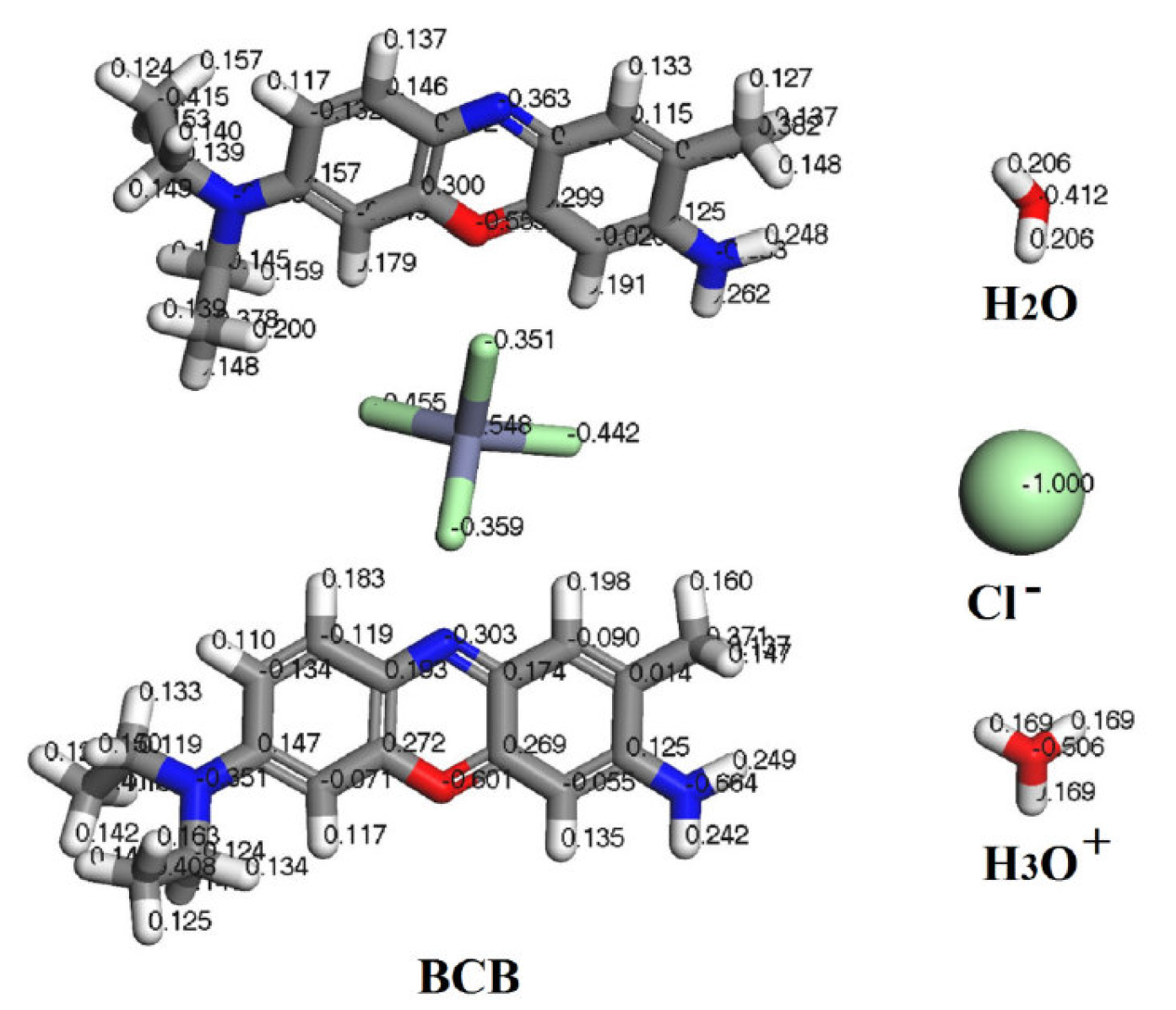
Figure 2 Optimized structures and Gasteiger atom charges assigned for the molecules used in the Monte Carlo calculations.
The MC calculations were implemented using 10 cycles of simulated annealing, with 100000 steps for each process. The temperature of the annealing process was set automatically from 105 to 102 K, for each cycle. Possible adsorption configurations were obtained as the temperature was slowly decreased.
Results and discussion
Fig. 3 presents cathodic and anodic polarization curves of a mild steel electrode immersed in a 0.5 M HCl aqueous solution, in BCB absence and presence, with different concentrations, at 298 K.
The inhibition efficiency IE (%) was calculated using the following Equation (1):
The electrochemical parameters, corrosion potential (Ecorr), Tafel slopes, polarization resistance and corrosion current density (icorr), were determined from the intersection of anodic and cathodic Tafel slopes. They are presented in Table 1.
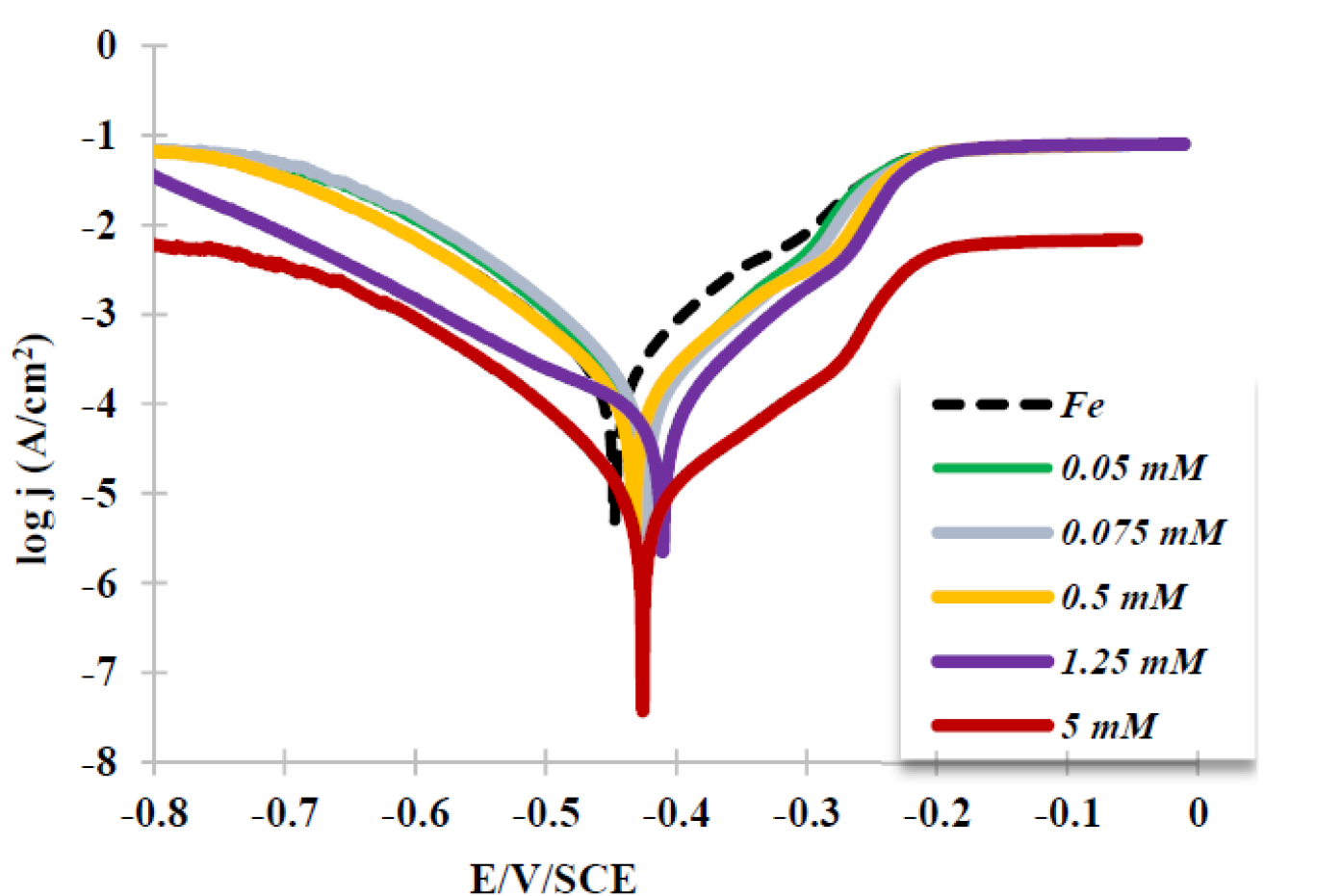
Figure 3 Mild steel electrode semilogarithmic polarization curves measured in an aqueous HCl solution (c = 0.5 M): in the absence and presence of 0.05 to 5 mM BCB.
Based on Tafel plots in Figs 1, it is obvious that BCB molecules adsorption onto the mild steel surface strongly depressed the mild steel corrosion current in this aggressive medium, showing a high corrosion inhibition efficiency value of up to 70.1 %.
Table 1 Inhibition efficiency, corrosion rate, Tafel slopes and polarization resistance of mild steel electrode in a 0.5 M HCl aqueous solution without and with BCB inhibitor, with different concentrations.
Corrosion media | Βa (V/dec) | βc (V/dec) | Ecorr (V) | Polarization resistance (Ω) | Corrosion rate (mm/year) | IE % |
---|---|---|---|---|---|---|
HCl | 0.099 | 0.084 | -0.447 | 926.76 | 2.764 | 0.00 |
+ 0.025 mM BCB | 0.088 | 0.081 | -0.431 | 939.84 | 2.594 | 6.13 |
+ 0.050 mM BCB | 0.087 | 0.073 | -0.428 | 1409.7 | 1.582 | 42.77 |
+ 0.075 mM BCB | 0.080 | 0.080 | -0.423 | 1441.4 | 1.556 | 43.72 |
+ 0.5 mM BCB | 0.093 | 0.089 | -0.432 | 1653.3 | 1.543 | 44.18 |
+ 1.25 mM BCB | 0.087 | 0.097 | -0.426 | 2044.5 | 1.259 | 54.47 |
+ 5 mM BCB | 0.161 | 0.069 | -0.411 | 3352.9 | 0.804 | 70.90 |
The polarization resistance (Rp) from Tafel extrapolation method was calculated through the use of the Stern-Geary Equation [21] Equation (2):
Table 1shows that, by increasing the inhibitor concentration, a larger polarization resistance was obtained, due to the inhibitor adsorption onto the metal surface, which efficiently passivated its active sites or created a physical barrier between the metal and the corrosive medium, enabling its corrosion protection [21, 22]. One can observe, during the anodic scan at the potential of about 200 mV more positive than corrosion potential, that the inhibitor adsorbed layer was removed.
Cathodic and anodic Tafel slopes (bc, ba) values slightly changed when the BCB molecule was present in the solution. These differences imply that the inhibitor affects the hydrogen evolution reaction kinetics n [6]. This corresponds to a higher energy barrier for proton discharge, resulting in less gas evolution. The studied molecules slightly changed the corrosion potential towards less negative values, acting as an anodic inhibitor. The increase in the polarization resistance confirms the corrosion inhibition ability displayed by the BCB molecule.
Monte Carlo results
The most stable adsorption configurations of BCB molecules onto Fe (1 1 0), obtained by simulated annealing using the Adsorption Locator module, are presented in Fig. 4.
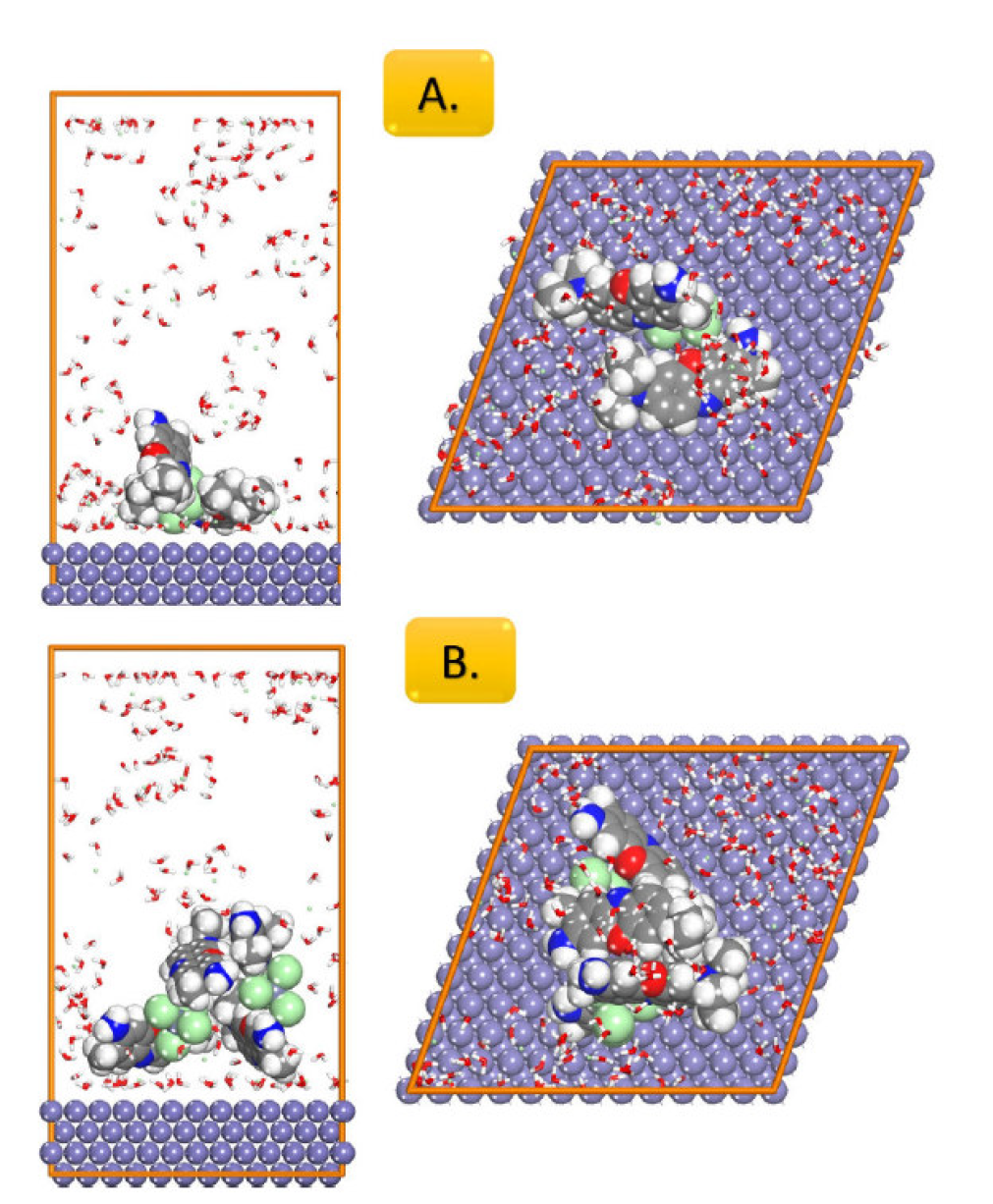
Figure 4 Top and side views of the most stable energy adsorption configurations for: A. One BCB molecule; and B. Two BCB molecules, in the corrosion media presence (150 water molecules, 20 hydronium ions, and 20 chloride ions) on the Fe (1 1 0) substrate attained by the adsorption locator module.
The BCB molecule adsorption, as shown in Fig. 4, is planar for one of the phenoxazin moieties, whereas the other moiety interacted only side-way with the surface. The planar adsorption was hindered by the tetrachloro zinc anion, due to its own tentative of surface adsorption. In the second case (when two BCB molecules were added to the iron slab), both phenoxazin moieties of the BCB molecules were side-way adsorbed onto the surface, and the aggregation of the second BCB molecule on the side of the other one was observed, revealing why there was only a minor difference in the adsorption energy, in the second case. Therefore, the BCB molecules ability to aggregate onto the metal surface explains the increased corrosion inhibition performance experimentally observed when BCB concentration was increased in the corrosion media.
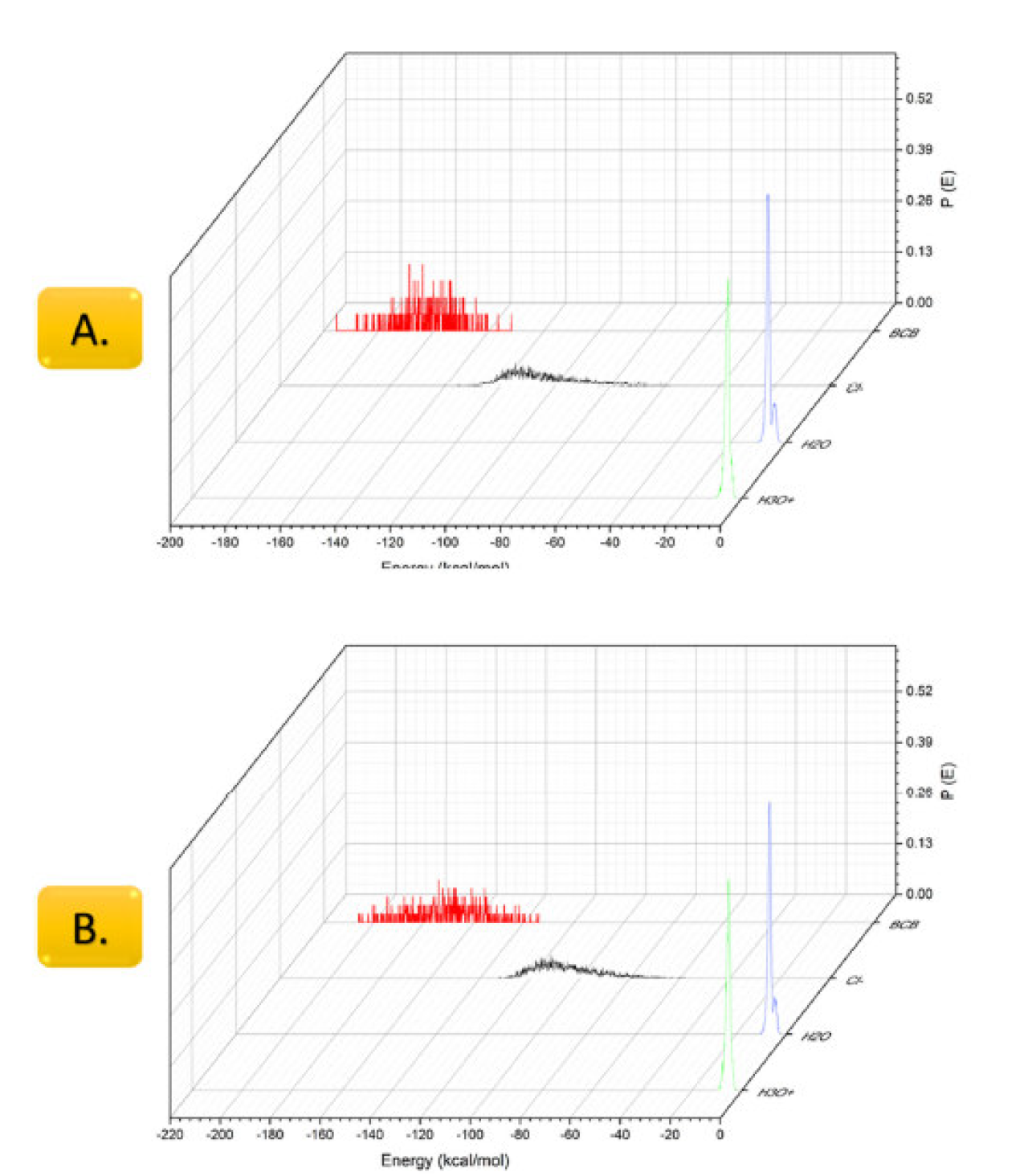
Figure 5 Adsorption energy distribution of the adsorbate: A. One BCB molecule and B. Two BCB molecules on Fe (110) surface, in the corrosion media (150 water molecules, 20 hydronium ions, and 20 chloride ions) presence.
The results of the adsorption energies during the Monte Carlo simulation are presented in Figs 5 A. and B. In the case of one BCB molecule per slab, its adsorption energy was in the range from -132 to -195 kcal/mol, with the maximum energy distribution probability value of -169 kcal/mol, whereas, when using two BCB molecules, the adsorption energy is -134 to -206 kcal/mol, with the maximum energy distribution probability value of -174 kcal/mol. The calculation predicts a high adsorptive tendency for chloride ions, in agreement with other data, as observed in many corrosion studies [23].
Inhibition mechanism
According to the bibliographic data, the corrosion inhibition mechanism in acidic media is well established. It describes the inhibitor molecule adsorption onto the metals surface by one of the four types of adsorption: (1) electrostatic attraction between charged molecules and the charged metal; (2) interaction of unshared electron pairs in the molecule with the metal; (3) interaction of π-electrons with the metal; and (4) a combination of the above [25]. A prerequisite of the physical adsorption process in the acidic medium is the presence of both a metal surface (with vacant low-energy electron orbitals) and charged species in the solution (a molecule having reasonably loosely bound electrons or heteroatoms with a lone pair of electrons).
The adsorbed BCB molecule may create a stronger interaction by coordinate covalent bond formation between electron pairs of N atoms of aromatic rings and the metal surface. An additional contribution to the adsorption ability is endorsed by the charged oxygen atoms of the phenoxazin moieties. These interactions lead to the formation of a protective film (with the interaction energy of -174 kcal/) on the mild steel surface, which reduces the corrosion rate, in accordance with the experimental results [25]. This formed organic film acts as a physical barrier, and prevents corroding species from reaching the metallic surface [16].
Conclusion
BCB inhibition efficiency has been explored experimentally and through the use of Monte Carlo calculations. Potentiodynamic measurements showed a meaningful decrease in the corrosion current, in the BCB molecules presence, and evinced a strong inhibition behavior of these moieties towards mild steel corrosion. The MC calculations allowed us to understand the fundamental reasons for this reduced corrosion rates. Experimental results show that the inhibition efficiency is dependent on the BCB concentration in the corroding media, reaching its maximum value at 70%. The MC calculations revealed that a high inhibitor concentration (BCB molecule) did not significantly change its adsorption energy, but led to a possible aggregation of the molecules.